INTRODUCTION
The Big Bang theory can satisfyingly explain the creation of the cosmos, but it fails to explain the interaction among various types of energy in the universe. Why did shortly after the Big Bang some elementary particles join to make protons and neutrons? And what made electrons bind to them later to create atoms? Why did these atoms then go on to build glaring stars and vibrant galaxies?
It turns out that all events in the universe can be blamed on four fundamental interactions (four fundamental forces) – gravity, electromagnetism, strong interaction and weak interaction. I am sure everybody has at least a basic overview of the first two forces, the last two, however, might be entirely foreign to some. But it is crucial to understand these interactions, since they govern the whole universe.
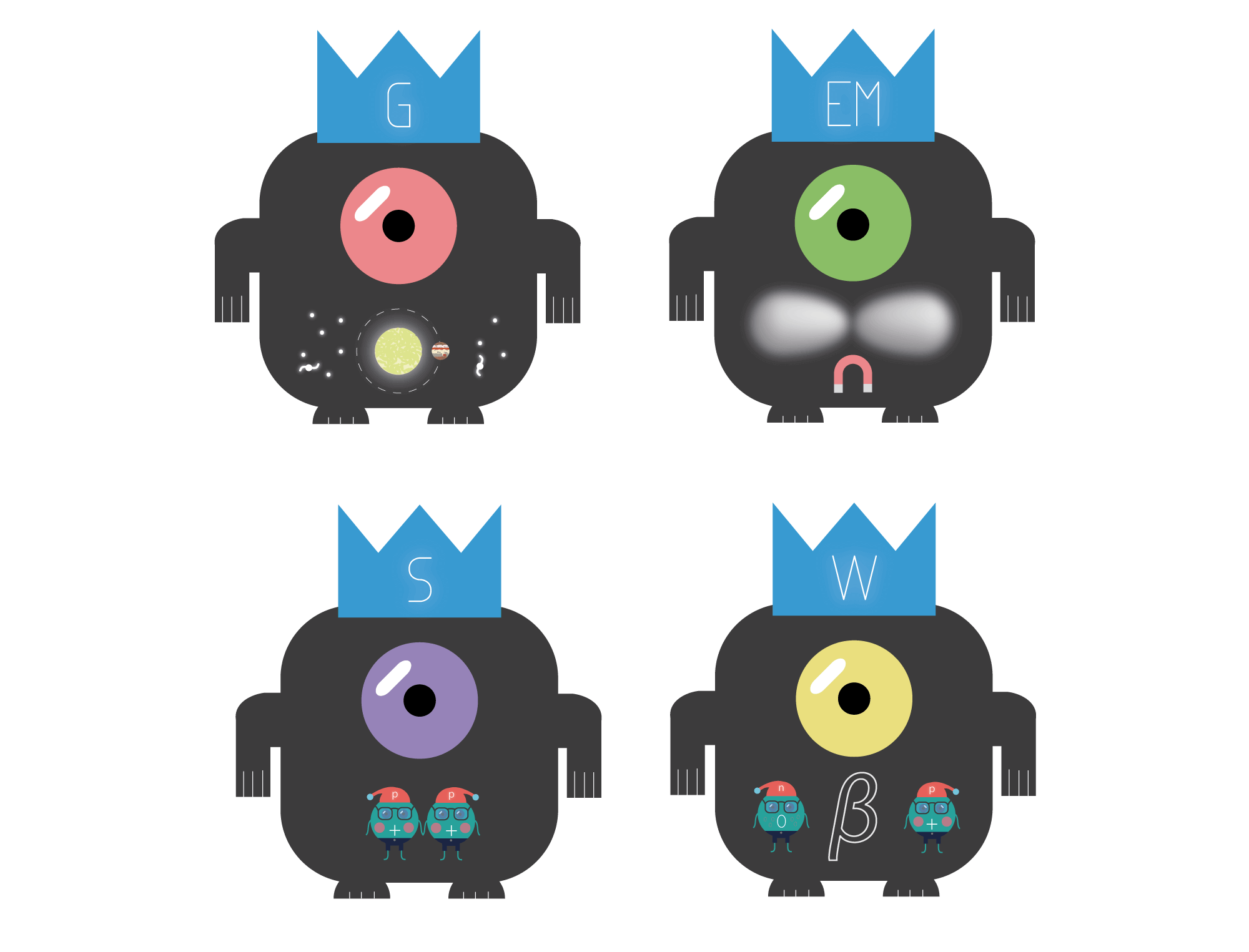
Take your own body as an example. First, let us dive deep into the microworld 1 , where we can see the basic building blocks of everything. Your body is, just like everything else in the universe, made up of energy. It is of course present in many various forms, but fundamentally, it is simply energy.
The energy of the human body is concentrated mainly in the form of elementary particles – the same particles that were created just a moment after the Big Bang. These particles then form composite particles – protons and neutrons. But what keeps elementary particles together? The answer lies in the strong interaction. If we jump one level up, we can see collections of protons and neutrons – atomic nuclei. Again, we can blame the strong interaction. Going another level up, we can see electrons, devoutly whizzing around the nuclei. Here, we observe the token of another fundamental force – electromagnetism. Individual atoms then go on to form molecules – electromagnetism shows itself once again.
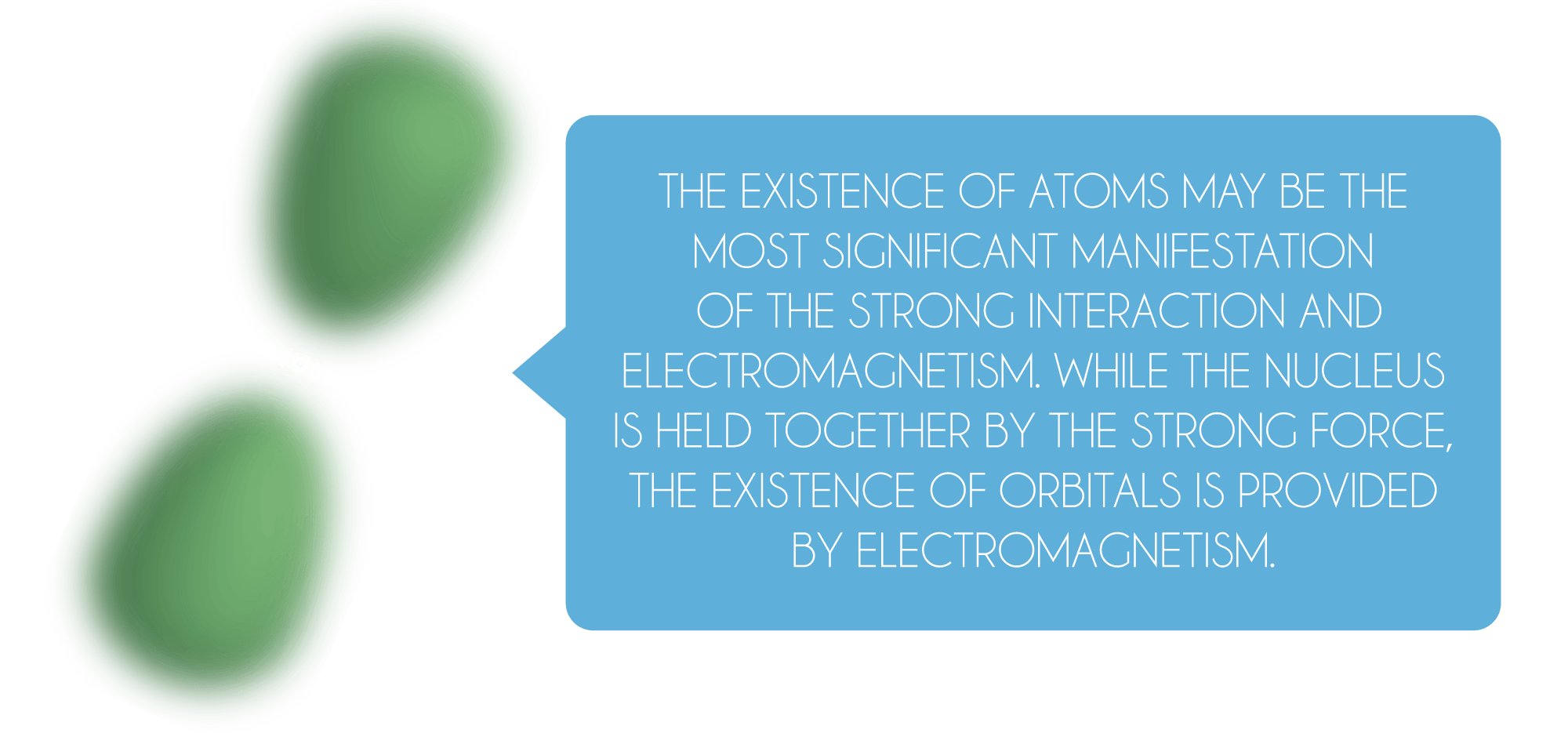
And finally, unless you are currently at the international space station or reading this text in a distant future on a faraway planet (most likely on Mars, as explained in one of the following chapters), it is quite likely that you are finding yourself on our tiny blue planet. And the only “force” keeping your feet on the ground instead of flying off to space is gravity – another of the four forces.
Our demonstration is over now. We have seen the essence of three of the four interactions using only the human body on Earth. If you are interested in the fourth force as well, you will have to wait a while – it manifests itself the least of the four forces. But now, let us analyse the interactions in detail, one by one. And we will start with the most sneaky and peculiar one – gravity.
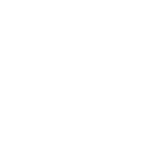
![]() |
NEXT |
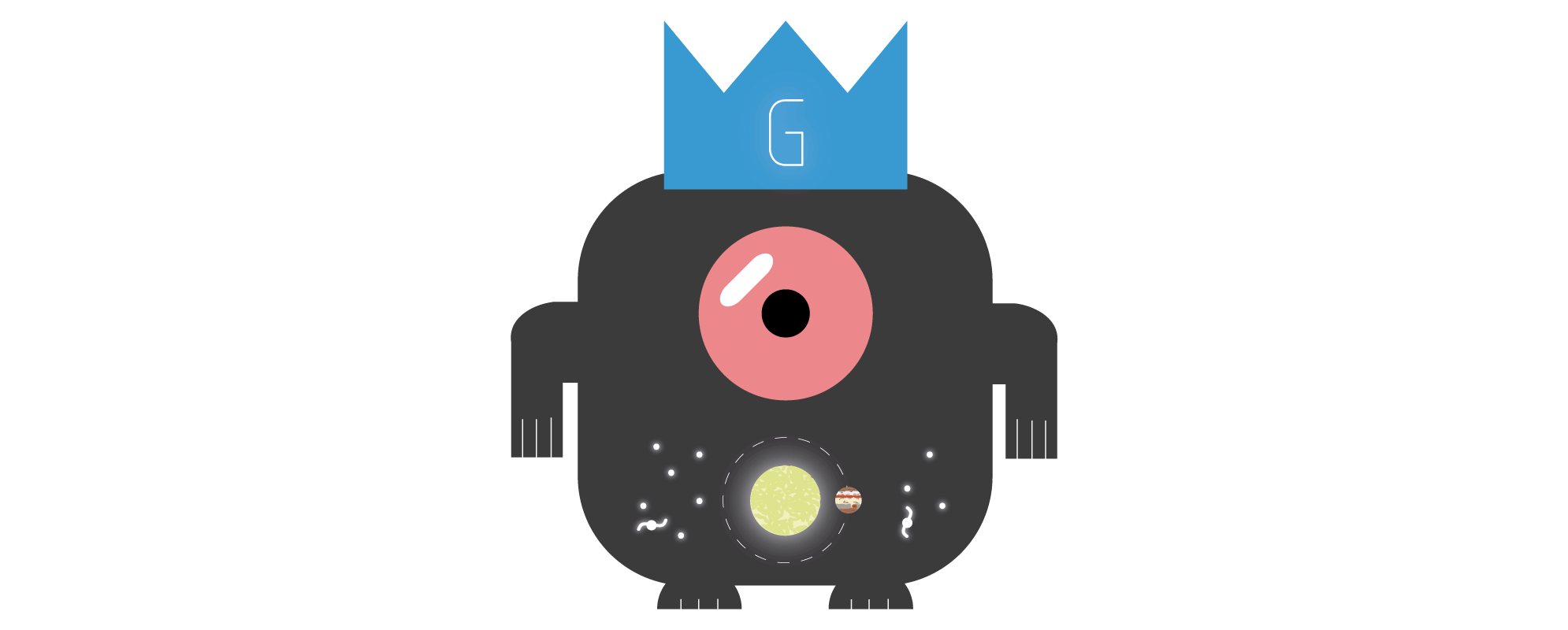
GRAVITY
Gravity differs from the other interactions in multiple ways. First of all, it is by far the weakest of fundamental forces. In fact, we can simply demonstrate this fact. Try to lift an object using your hand. A pencil, a glass, anything. If you have succeeded and the object is safely in the air surrounded by your palm, congratulations – you have just managed to overcome the gravitational pull of the entire Earth, whose mass is in trillions of trillions of kilograms. How can gravity be the dominant force of the universe when it is so immensely weak?
The reason is that the other three interactions, though much stronger, simply are not customized to become the prevailing force of the universe. Strong and weak forces have a very short range – they only affect objects that are far less than a billionth of a meter apart. And the last interaction, electromagnetism, only influences objects with an electric charge. The problem is that you do not find such objects very often in the macroworld – most objects are neutrally charged. So the only reason that this ridiculously frail interaction has become the motive force of the cosmos is that it simply has no competition.
The second factor that makes gravity special is that it is presumably not really a force, even though it has been viewed as such to the beginning of the 20th century. However, with the advent of Einstein’s theory of relativity, our view of gravity has changed radically. Einstein saw gravity merely as a curvature of space-time. Every object in the universe simply creates a kind of dimple in the space-time continuum and all other objects are inclined to move closer to that object.
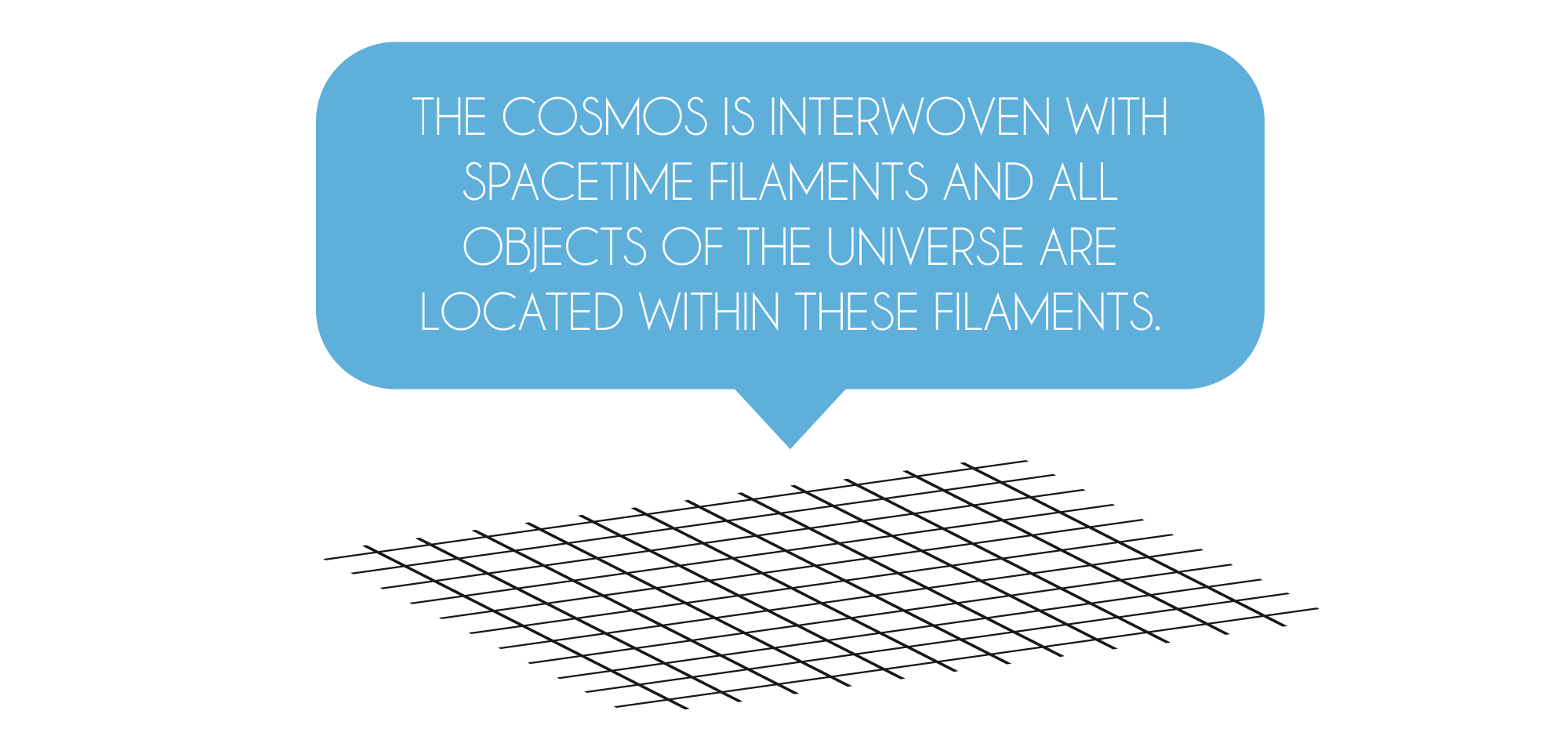
It is like placing a heavy object into the middle of a trampoline – the entire surface of the trampoline curves downwards, and if you place a different object near its rim, it starts to roll towards the original object. This analogy, however, has an imperfection. Just like with the inflation of the universe after the Big Bang, we need to take away one dimension to comprehend the phenomenon.
The surface of a trampoline can be perceived as two-dimensional space (it has width and height, but no depth) similarly to a sheet of paper. An object placed to its middle causes its two-dimensional space to curve. Therefore, the surface of a trampoline with an object in its centre can be understood as a two-dimensional space curved in the third dimension.
However, our universe is three-dimensional, so any curvature caused by the presence of an object in our space-time occurs in the fourth dimension. That is also the reason why we can never perceive any gravitational curvature. We would need to be four-dimensional beings for the curvature to be revealed to us.
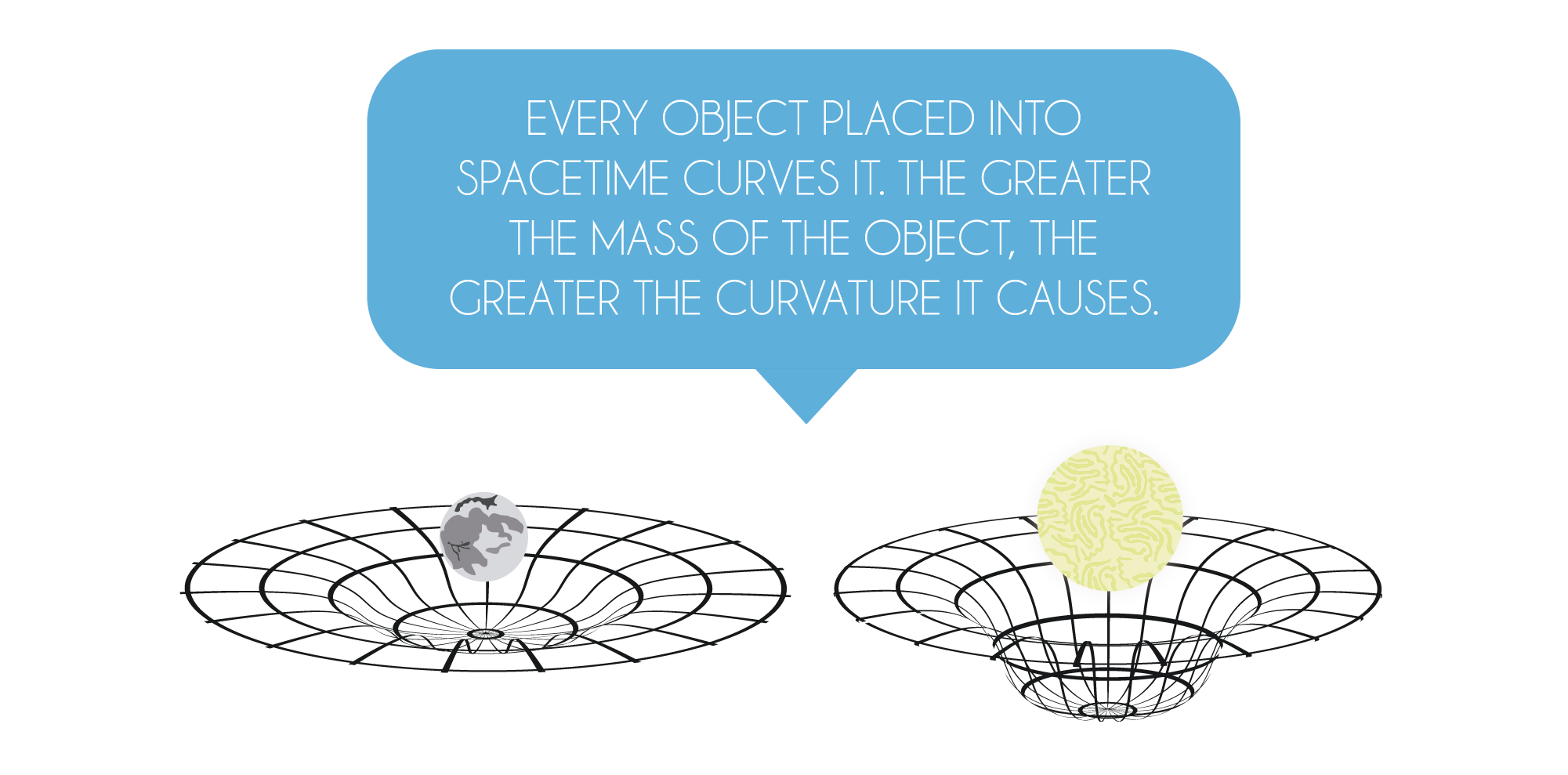
However, it does not hurt to know that nobody is sure whether this theory of gravitational space-time curvature is true. With today’s technical advancement, we are struggling to find evidence that gravity indeed curves our three-dimensional space.
But there is another view of gravity, completely different from the one I have just described. According to this view, gravity is provided by a hypothetical particle called the graviton. How? Simply said, every two objects in the universe exchange various numbers of gravitons, which causes them to attract.
To understand why there are two different perceptions of gravity today, we first need to become acquainted with the greatest problem of today’s physics – the everlasting search for the theory of everything. To achieve that, we need to travel more than a hundred years to the past, to the beginning of the 20th century, where we will witness the birth of the two greatest physical theories of today.
By the end of the 19th century, some physicists presumed that physics was already complete. They thought that everything had already been described by the old physical theories. But then came the year 1900, along with a new revolutionary theory called quantum mechanics, which proved how immensely wrong those physicists were. This theory describes the behaviour of objects from the microworld, which is completely different form the behaviour of “normal” objects. 2 Fifteen years later, classical physics was stabbed again by Einstein’s general theory of relativity, which utterly transformed our view of gravity and beautifully described the motion of objects at high velocities.
However, there is a tremendous problem with these two theories – each one seems to describe a completely different world. While quantum mechanics successfully uncovers the peculiarities of the microworld, general relativity brilliantly describes the motion of objects of the macroworld. But if we wish to fully comprehend our mysterious universe, we need to unify these two incompatible theories into one. Physicists have been trying to achieve that for the past hundred years, so far without much success. 3
And the problem with today’s view of gravity rises from here. While the description of the remaining three interactions comes from quantum mechanics, the best understanding of gravity is provided by general relativity. Physicists therefore aim to describe gravity within the framework of quantum mechanics, so that it forms a single integrated theory. This non-existent theory is called the theory of quantum gravity or simply the theory of everything.
And that is the reason why there are two different views of gravity today – one almost perfect in the framework of general relativity, which is not compatible with other interactions, the other not so perfect within quantum mechanics, which is crucial for the upcoming theory of everything, but includes these peculiar particles called gravitons, which have never been detected.
Not to worry though – luckily, there are a few things that we know about gravity with certainty. Firstly, gravity is always attractive. There is no instance of two objects gravitationally repulsing each other. Secondly, gravity propagates with the speed of light, which is the highest velocity anything can reach when traveling through space-time. 4 That means that if the Sun were to disappear now and stop influencing us gravitationally, it would take exactly 8 minutes and 20 seconds for us to notice it and free ourselves from the Sun’s gravitational field (at the same time, the Sun would also disappear from the sky, as the last of its light would reach our planet). Until then, the Earth would keep revolving around the non-existent Sun.
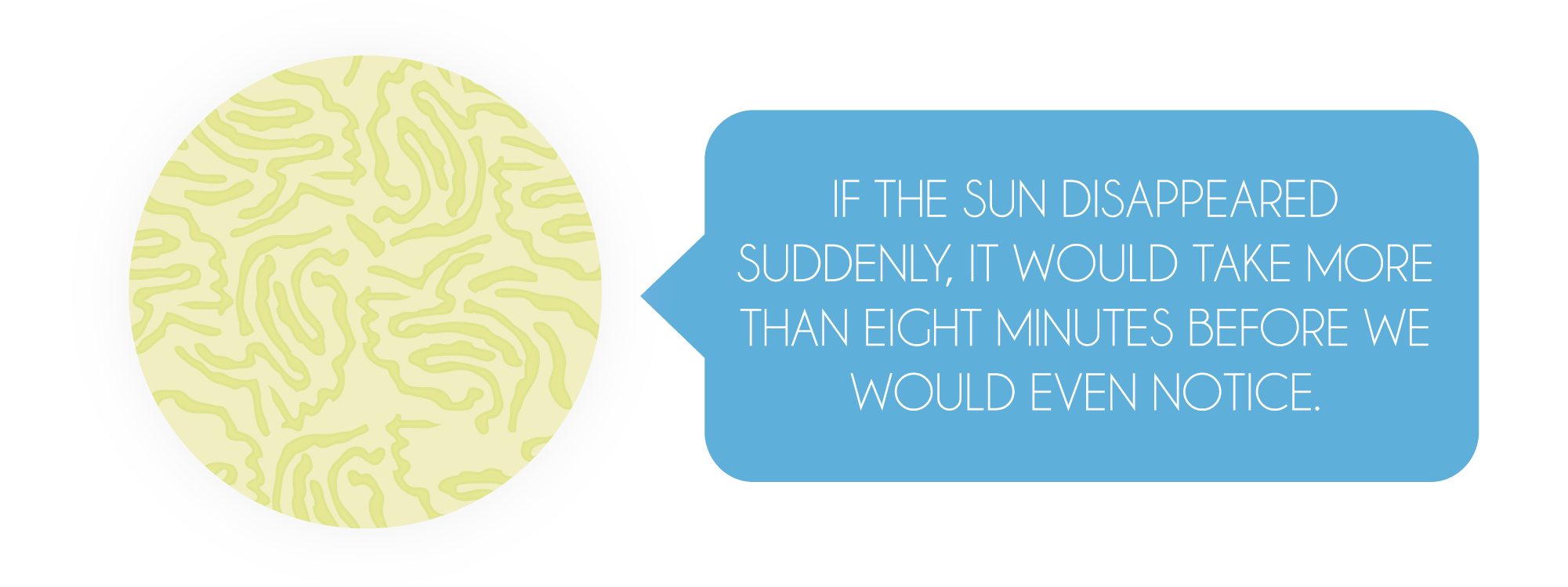
And the third fascinating thing we know about gravity is that its range is infinite. Your own body attracts all the other objects from the observable universe – though it may seem peculiar, you are gravitationally attracting your computer, every single person on this planet, or the Andromeda galaxy, located several million light years away. 5 It goes without saying that the gravitational interaction between you and the objects around you is absolutely negligible – gravity is simply too weak and its power starts showing only with overwhelmingly huge objects.
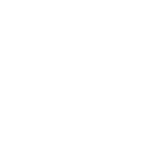
![]() |
![]() |
![]() |
PREVIOUS |
SHARE |
NEXT |
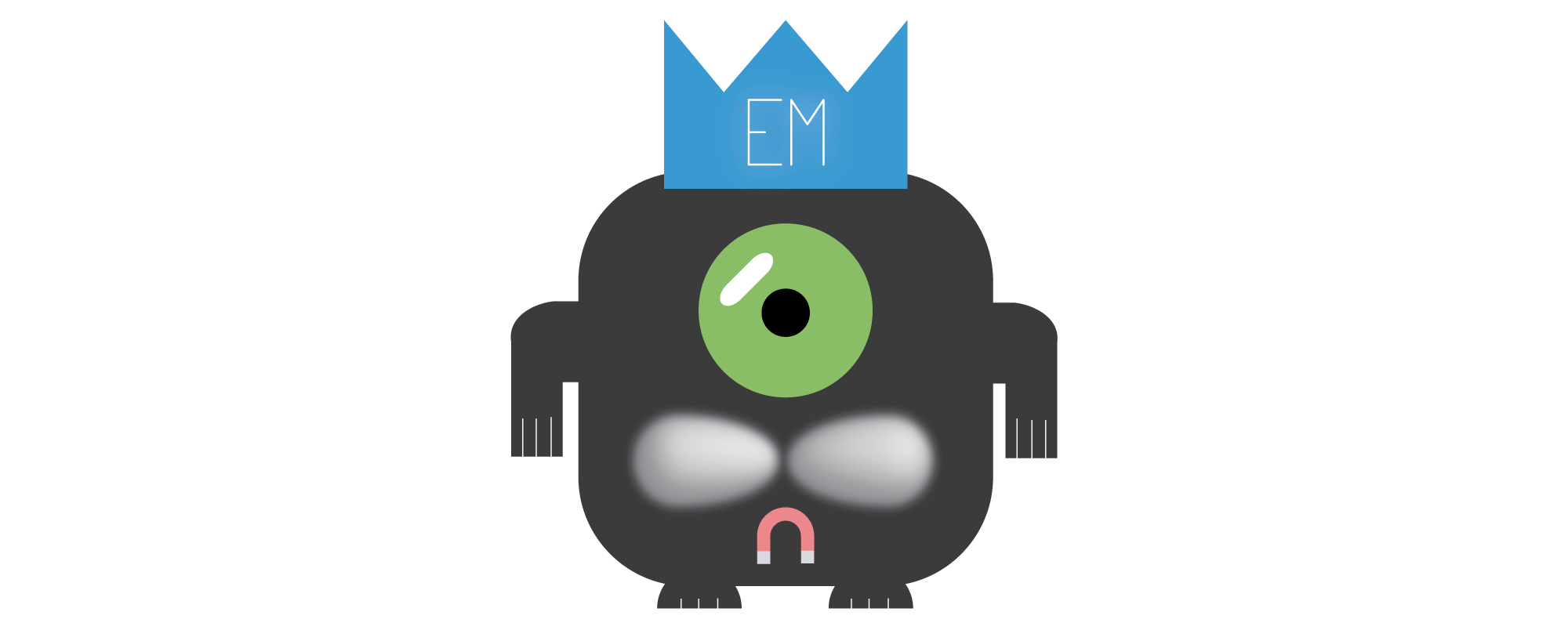
ELECTRICITY AND MAGNETISM
Electromagnetism might be the best-known interaction to us. However, we mostly speak about two separate entities. First of them, electricity, is a force our entire modern society is based upon, since it propels possibly all modern devices, from the most primitive calculators to the fastest supercomputers. Without electricity, we would never have televisions, the internet, computers, and a lot more. There is no doubt that electricity is crucial for the proper functioning of today’s society. But what about the other of the two entities? Magnetism is mostly known for being an endearing force, due to which we can attach various objects to our fridges. But is electromagnetism really like that?
It turns out that electricity and magnetism are very closely related. So related, in fact, that one could not exist without the other. If you move an electrically charged particle (electron, for instance), a magnetic field is spontaneously formed around it. Conversely, anytime you move a magnet, an electric field is created. This phenomenon is exploited in power stations. Nearly all power stations are built on the principle of electromagnetic induction – a magnetic field is placed to the vicinity of an electrical conductor and spins perpetually (here, different types of power plants come into play – each type achieves the spinning of the magnetic field in a different way), which causes electric current to form in the conductor.
Just like gravity, electromagnetism has an infinite range. Unlike gravity, however, it does not affect every single object in the universe but only objects with electric charge (opposite charges attract each other, same charges repel). Nevertheless, electromagnetism can be observed all around us. Let us now describe individual manifestations of electromagnetism one by one. And we are going to start somewhat unexpectedly – with sight.
Sight is the most important of human senses. More than three quarters of all sensory perceptions our brain receives to process are provided by our eyes. Eyes that have been through hundreds of millions of years of evolution, so that they can function properly. All the various organs of sight, from the simplest of eyespots inside the bodies of minuscule organisms, to the most perfect eyes in the animal kingdom 6 , have the same mission – to detect a specific part of the electromagnetic radiation which we like to call light.
As the name itself implies, electromagnetic radiation has something to do with electromagnetism. Its creation and propagation occur quite simply – a moving electric field generates a magnetic field, which in turn again generates an electric field, and so on. These repeating fields travel through space-time (at the speed of light, of course) until they reach an impediment that would absorb them.
For example, the electromagnetic radiation sent by the screen of the device on which you are reading these words travels into your eye, where it is absorbed and transformed into electric signals leading right to your brain. Once there, the signals are processed and consequently decoded as an image. If electricity and magnetism were not a conjoined entity, light would not be able to exist.
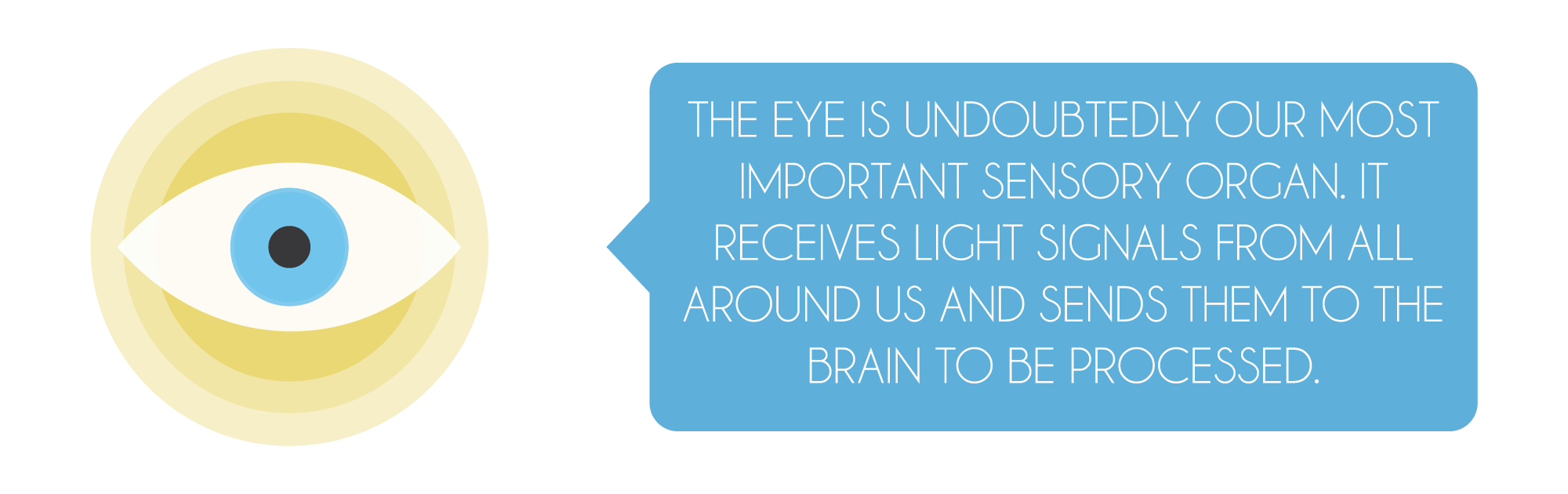
Now let us move to the subatomic level. Focus on any object in your field of vision. Positively charged protons attract the negatively charged electrons, which ensures the existence of the atoms your chosen object is made of. If we move a level up, we quite likely encounter molecules (collections of atoms) of the object. These molecules are formed due to diverse chemical bonds, which again are a manifestation of electromagnetism. 7 In short, if this mesmerizing interaction ceased to exist, all objects around you – including yourself – would immediately break up into individual subatomic particles.
As we have just demonstrated, electromagnetism is, just like gravity, crucial for the existence of the cosmos as we know it. The manifestations of this interaction could be discussed much longer. However, we are going to move to the next, no less important force – the strong interaction.
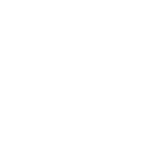
![]() |
![]() |
![]() |
PREVIOUS |
SHARE |
NEXT |
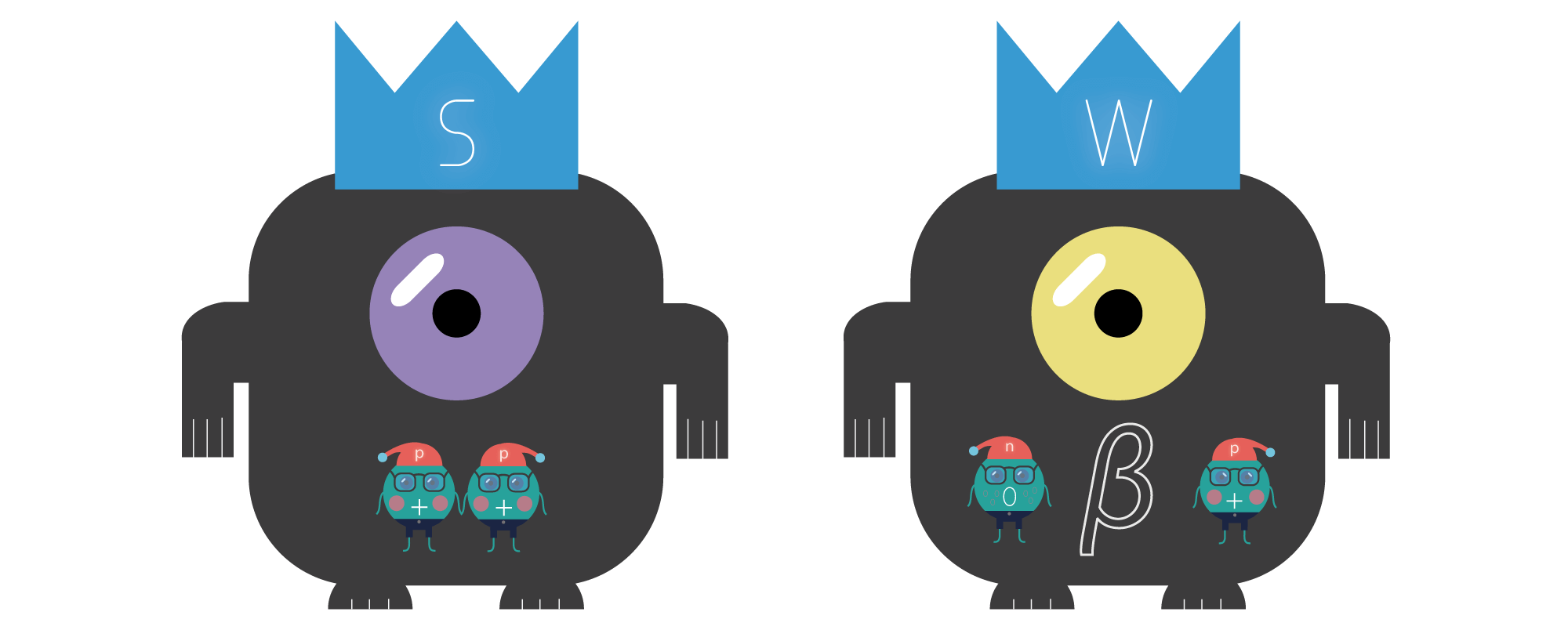
STRONG AND WEAK INTERACTION
The strong interaction is, as suggested by its name, the strongest of all interactions. It is about a hundred times stronger than electromagnetism (and about a hundred trillion trillion trillion times stronger than gravity). How is it possible that we almost never hear about this interaction if it has such an immense strength? The problem is that its range is just billionth of a millionth of a meter. It may seem that a force with such a short range would never be able to influence our universe in a significant way, but the truth is that without the strong interaction, humans would never be able to exist.
As already mentioned, the universe was flooded with elementary particles right after the Big Bang. Those then started clumping together to create composite particles – quarks started forming protons and neutrons. But what caused them to attract? Why were quarks so keen on creating more complex particles? As you may already suspect, the attraction between individual quarks was provided by the strong interaction.
It does not end here, though. The strong interaction is to blame for another crucial phenomenon of our reality – the existence of atoms. We already know from the previous chapter that the same electric charges repulse each other. However, this means that protons in the nuclei of complex atoms should repulse and escape into all conceivable directions. But the strong interaction ensures that protons remain together. If electromagnetism were just a tiny bit stronger than the strong interaction, the existence of atoms would simply be impossible.
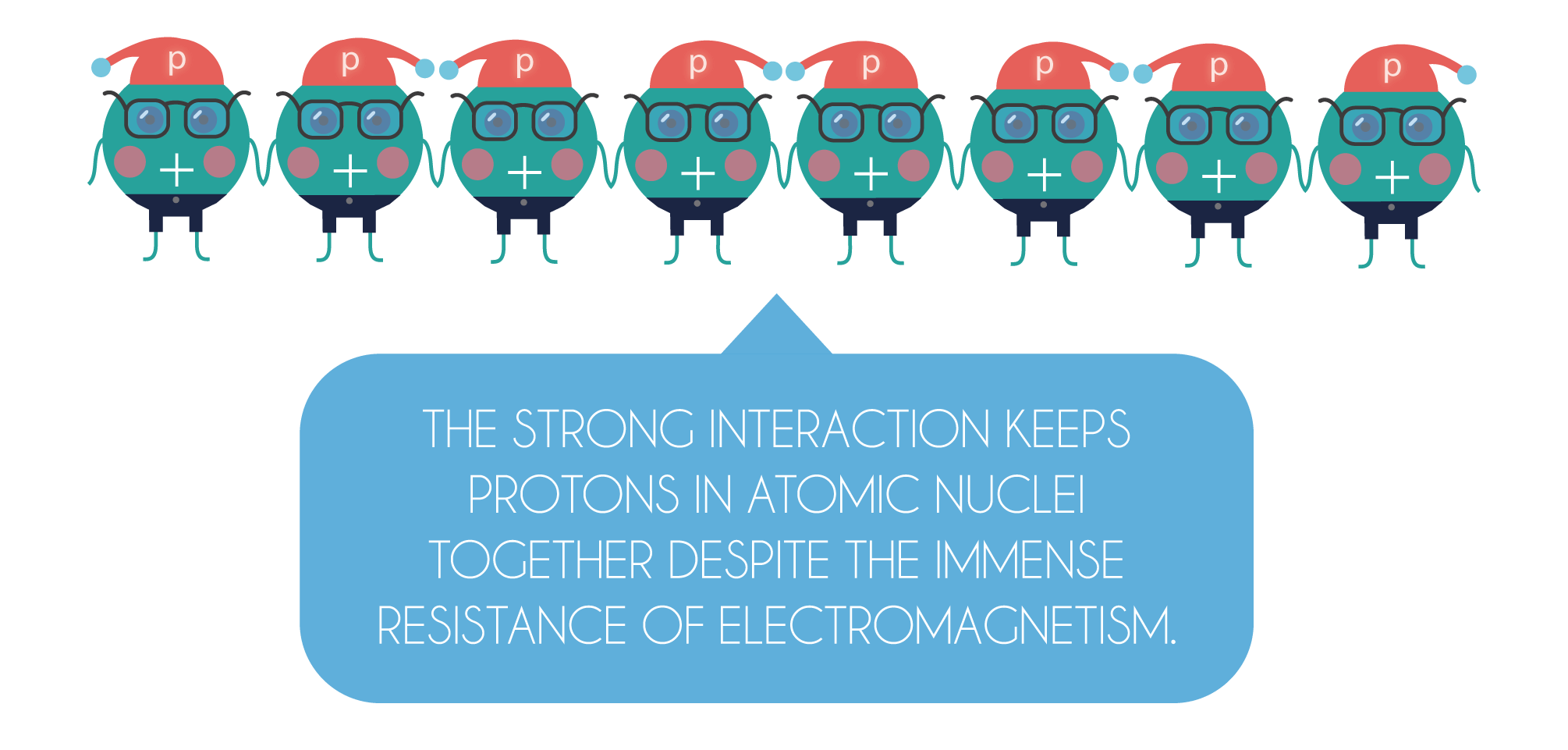
The final force, the weak interaction, may be the least known and the least interesting of all interactions. However, this is not to say it is not important. The weak interaction has the power to turn a neutron into a proton. Why would it do that? Within some atoms, there is an unstable ratio between the number of electrons and the number of protons in the nucleus. And the weak interaction is here to make sure that this instability is eliminated. When there are too many neutrons in a nucleus, the weak force simply turns one of them into a proton. This phenomenon is called the beta decay.
As we know from the first chapter, the number of protons in the nucleus determines what atom we are dealing with. But this means that any time an atom undergoes beta decay, which adds one proton into its nucleus, the whole atom changes into a different element. The weak interaction can therefore turn carbon into nitrogen or hydrogen into helium just by turning neutrons into protons.
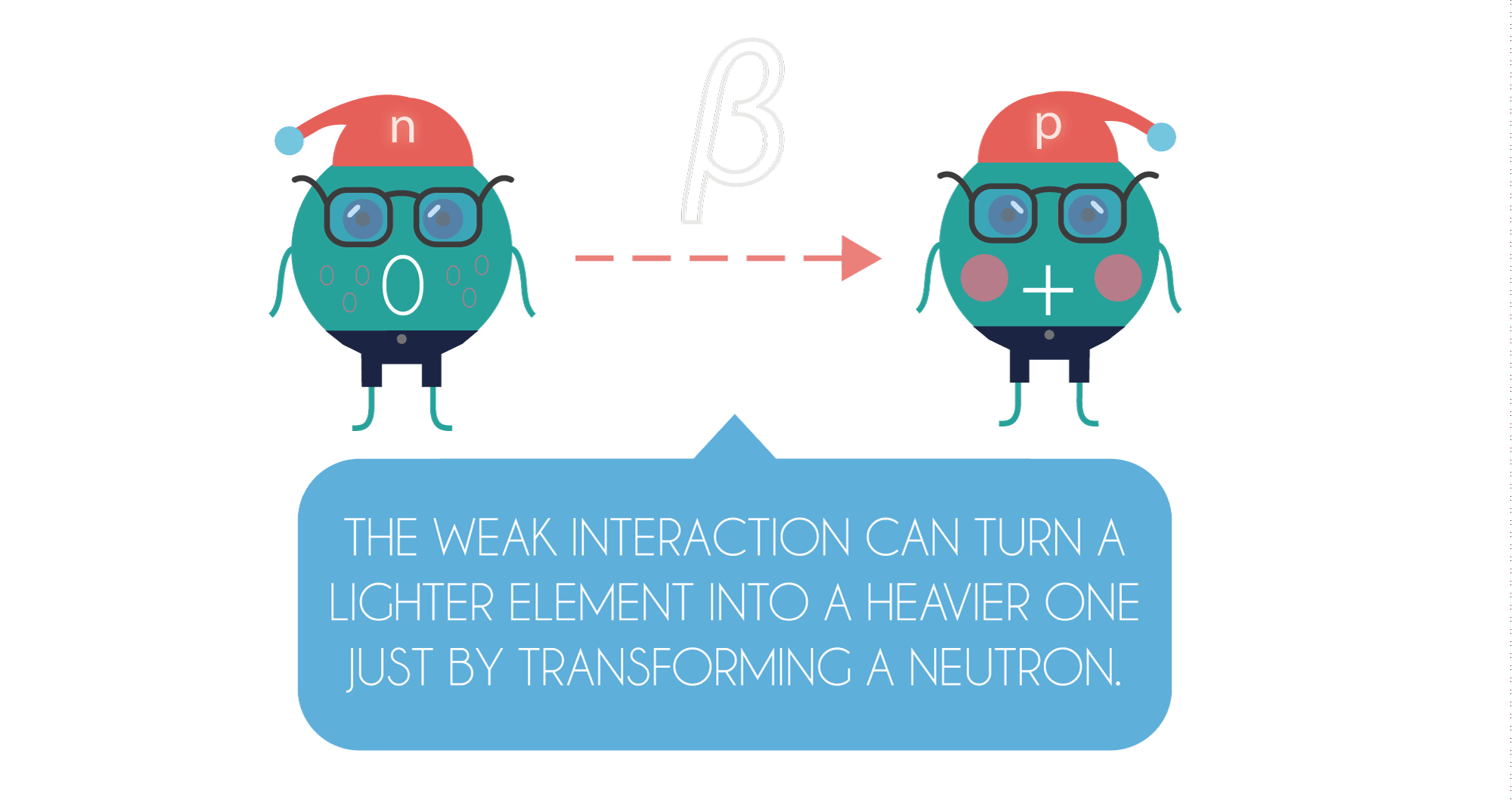
And that is all. We have reached the end of the story of interactions. They make sure that our universe functions the way it does and without them, we would have never existed – perhaps with the exception of the weak interaction, which is the only one that does not affect the course of the cosmos in a significant way. Now, we can focus our attention on another fascinating element of our universe – huge fusion factories which are making billions of photons each moment.
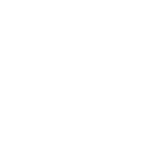
![]() |
![]() |
![]() |
PREVIOUS |
SHARE |
NEXT |