INTRODUCTION
By all accounts, we seem to live in a universe whose arrow of time only goes in one direction – forward. Whether we like it or not, adventurous journeys into ancient history may forever remain a mere figment of human imagination. But what if we had the opportunity to travel back in time? We could live through yesterday or the year 2000. We could participate in the Second World War, spectate the development of first civilisations, or even observe the formation of our own planet. But how far would we be able to go? If our universe were static and eternal, we could travel as long as we would like. But from what we know, we do not live in such a universe. That is to say, on your journey, we would eventually arrive at the very beginning of time.
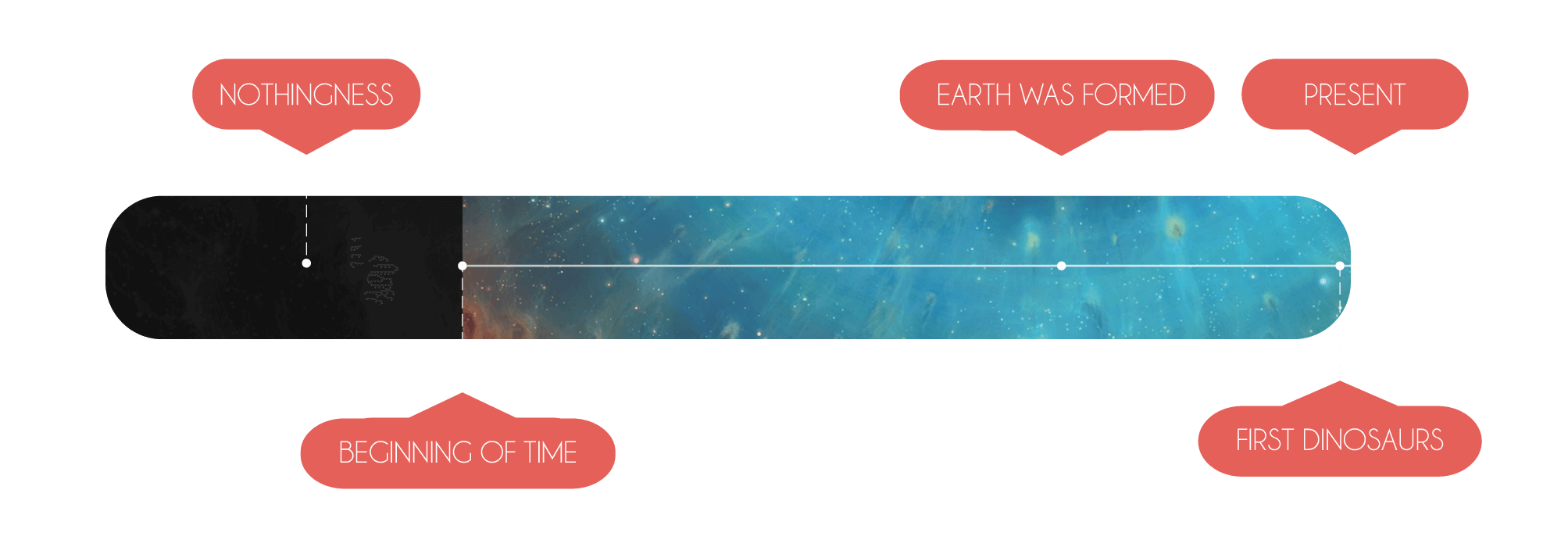
However, if we want to accept the theory that our universe had a beginning, we should focus on one crucial question – what was the universe formed from? Unfortunately, nobody knows the answer to this question yet, and there is a possibility that it is going to stay this way forever. But there are two options.
The first possible answer is that the universe was simply created out of nothing . It is not a great surprise that this idea, which defies both fundamental laws of physics and common sense, is not very likable. The second and a much more interesting option is that our universe was created because of a certain impulse. What impulse? Today, nobody knows. In the future, we might reach sufficient technological advancement that we will be able to study such an impulse. But right now, we can only argue and devise hypotheses. Perhaps the creation of our cosmos was caused by a collapse of a different (prior) universe, and thus we are just a link in an infinite set of universes, each one being created when the previous one dies. Perhaps the universe was born in a collision of two different universes. Perhaps.
Even though we do not know the answer to this great question, there are certain things we do know about the evolution of the cosmos. Or – to be scientifically accurate – there are some things that we suspect. The essence of science is trying to understand the world around us as well as we can. We may achieve that by constructing hypotheses and authenticating them using the scientific method.
Anybody can devise a hypothesis. We can construct a simple thought experiment as an example – a prehistoric man sees strange dots in the night sky (today known as stars) and draws a conclusion that somebody must have painted them on the firmament – he has just created a hypothesis. To check the hypothesis, the prehistoric man builds a rocket (let us neglect the fact that the technical advancement of prehistoric people, whose apogee is knowing how to make fire, is nowhere near the level necessary to construct something as immensely sophisticated as a rocket) and sends this rocket far away, so that it can meet those mesmerizing dots – he is authenticating his hypothesis using the scientific method. Much to his dismay, the rocket is serenely wandering through space with no firmament in sight. His hypothesis has just been disproved.
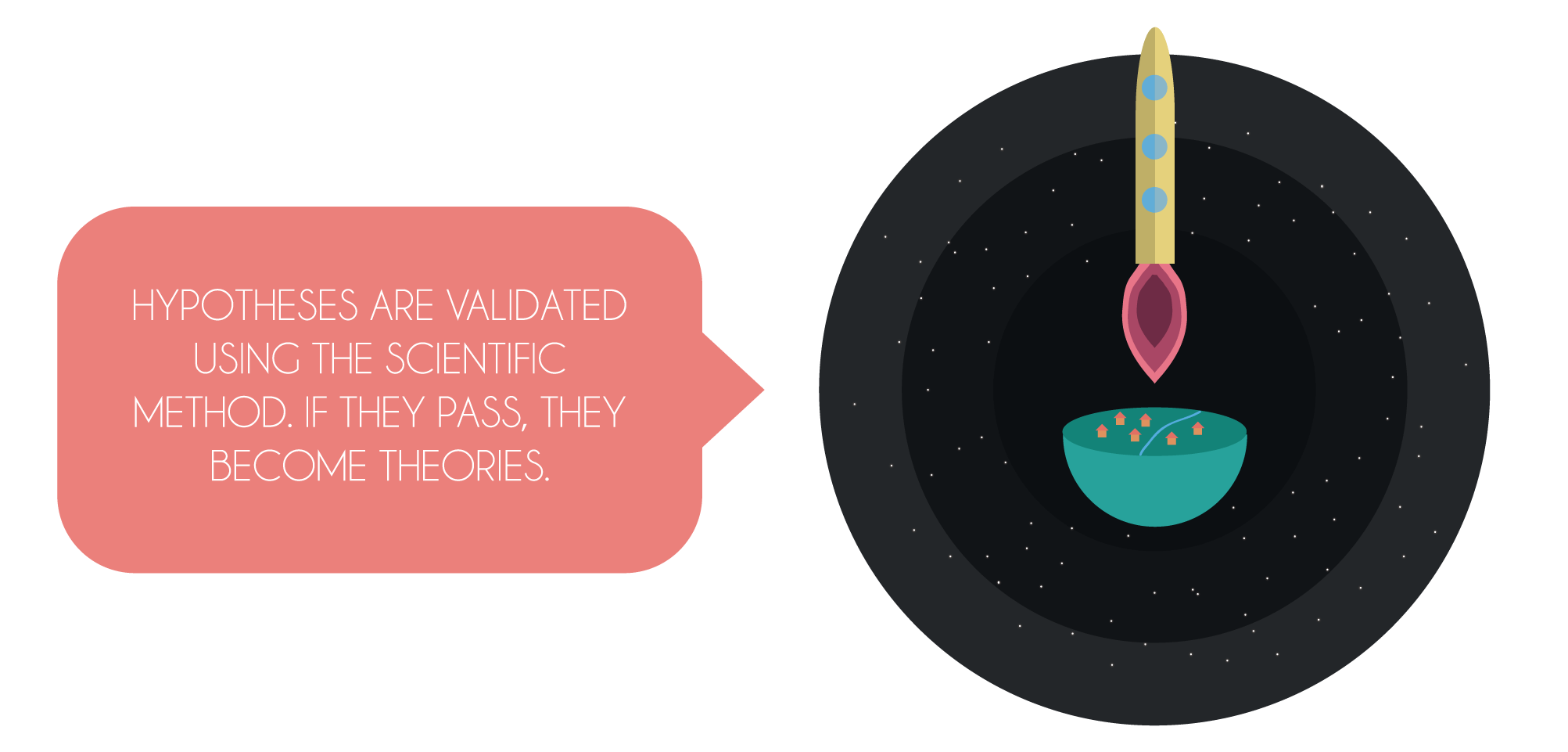
However, if a hypothesis does pass all trials we impose on it, it is promoted to a theory. Now, we are finally talking about science, which is – in its purest form – a simple collection of such theories. Whether we are dealing with physics, psychology, biology, chemistry or astronomy, this element is common for all sciences. The entire human knowledge is just a large pile of theories, which are categorized into individual branches of science.
But there is another very important factor at play – some people consider theories an absolute, unshakeable truth. This view is incorrect. Science perceives theories merely as a collection of fragments, each of them trying to describe a tiny piece of reality. Some fragments are very precise, others not so much. If a theory is found insufficiently accurate, scientists are immediately ready to replace it with a different, better one. A good example is the multitude of theories which are trying to describe one of the most common phenomena in the universe – gravity. For many years, the best description of this mysterious entity by far was provided by Newton’s theory of gravity. However, it has been found somewhat inaccurate in some extreme cases. Eventually, it has been overthrown by Einstein’s general theory of relativity.
However, it was not such a long time ago that scientists realised that even general relativity is not perfect – it is not able to describe the gravitational interaction at the submicroscopic level. Unfortunately, no valid alternative has been found yet and general relativity is still awaiting its successor.
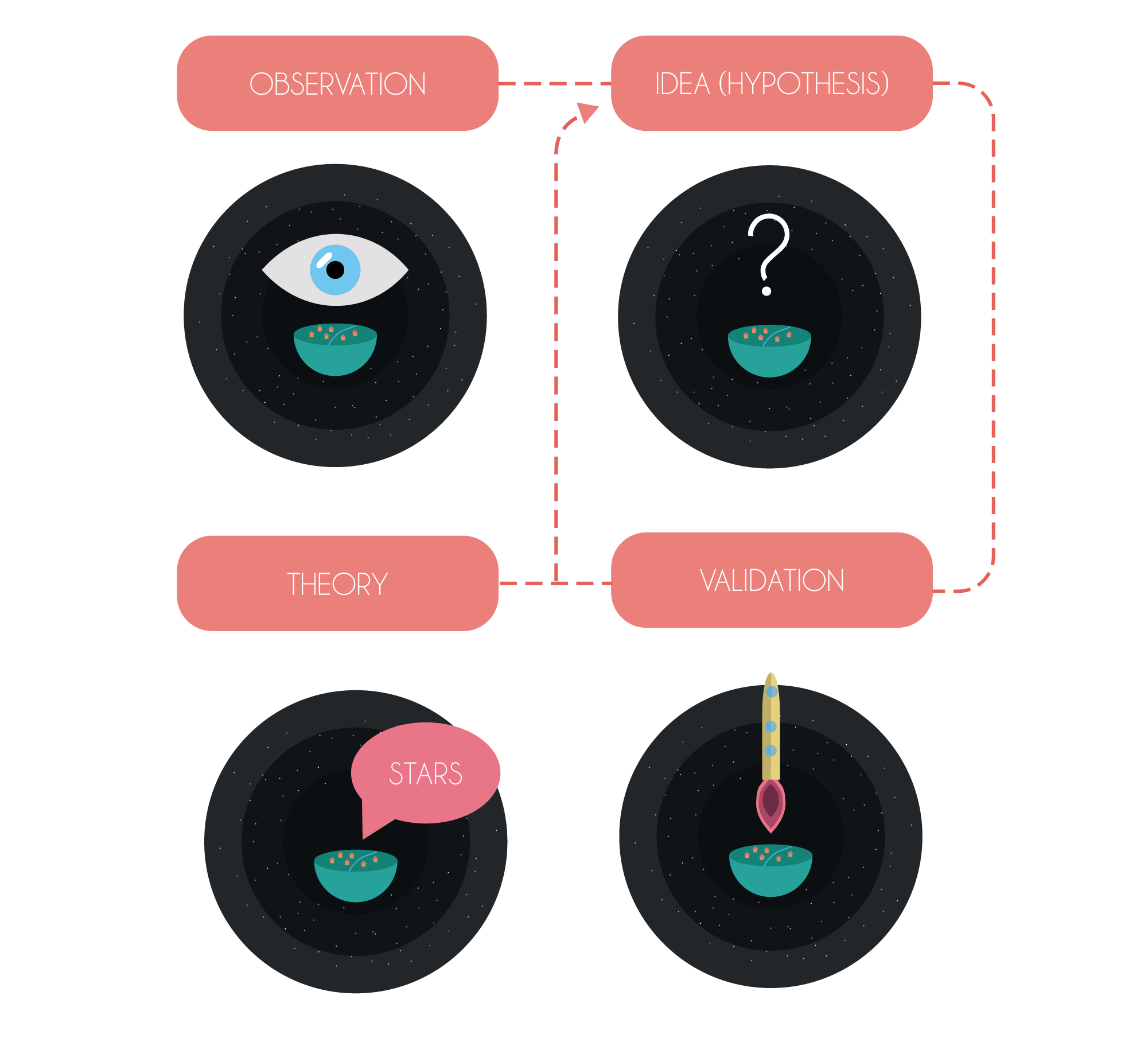
This example shows us the cruel truth about science – we can never be sure of anything. Even though we might think that we have revealed some tiny piece of reality, there is always a possibility that we have neglected a certain aspect of the cosmos in one of our theories, and thus something completely unexpected might happen. Who knows? Perhaps there is a space constant – whose existence is hidden from us – which will annihilate all of us. Perhaps the universe will die today.
There is nothing to worry about though. It is pointless to think about such things, since we are again finding ourselves in the treacherous world of hypotheses. Let us go back into the scientific world of theories, which still provides by far the best description of reality – despite its potential drawbacks. Specifically, let us devote our attention to a great theory that provides the best summary of everything we suspect about the creation of the universe itself – the Big Bang theory.
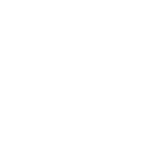
![]() |
NEXT |
THE BEGINNING OF EVERYTHING
At the very beginning, there was a peculiar object called the singularity, floating in nothingness. How did it get there? That is a mystery. However, before we start exploring this immensely interesting object, we should focus on a different, seemingly trivial expression – nothingness.
Some might think that all the “empty” space around us could be considered nothingness. But that can hardly be the case, given that each centimetre of air contains billions of atoms.
Others might argue that the vacuum of space could be classified as nothingness. After all, vacuum is empty by definition – it contains no matter whatsoever. But there is a catch – vacuum is inside the universe just like everything else around us. And the universe is interwoven with space-time filaments. But space-time certainly is something. It is a specific area where all the laws of our universe are in effect.
For this reason alone, one cannot consider vacuum as complete nothingness, not to mention the immense amount of particles that are being created each second in every single tiny bit of vacuum as a result of quantum mechanics. 1
But the nothingness around our initial singularity was special and unique – time and space were non-existent and the laws of physics powerless. We would never be able to find such nothingness in our cosmos.
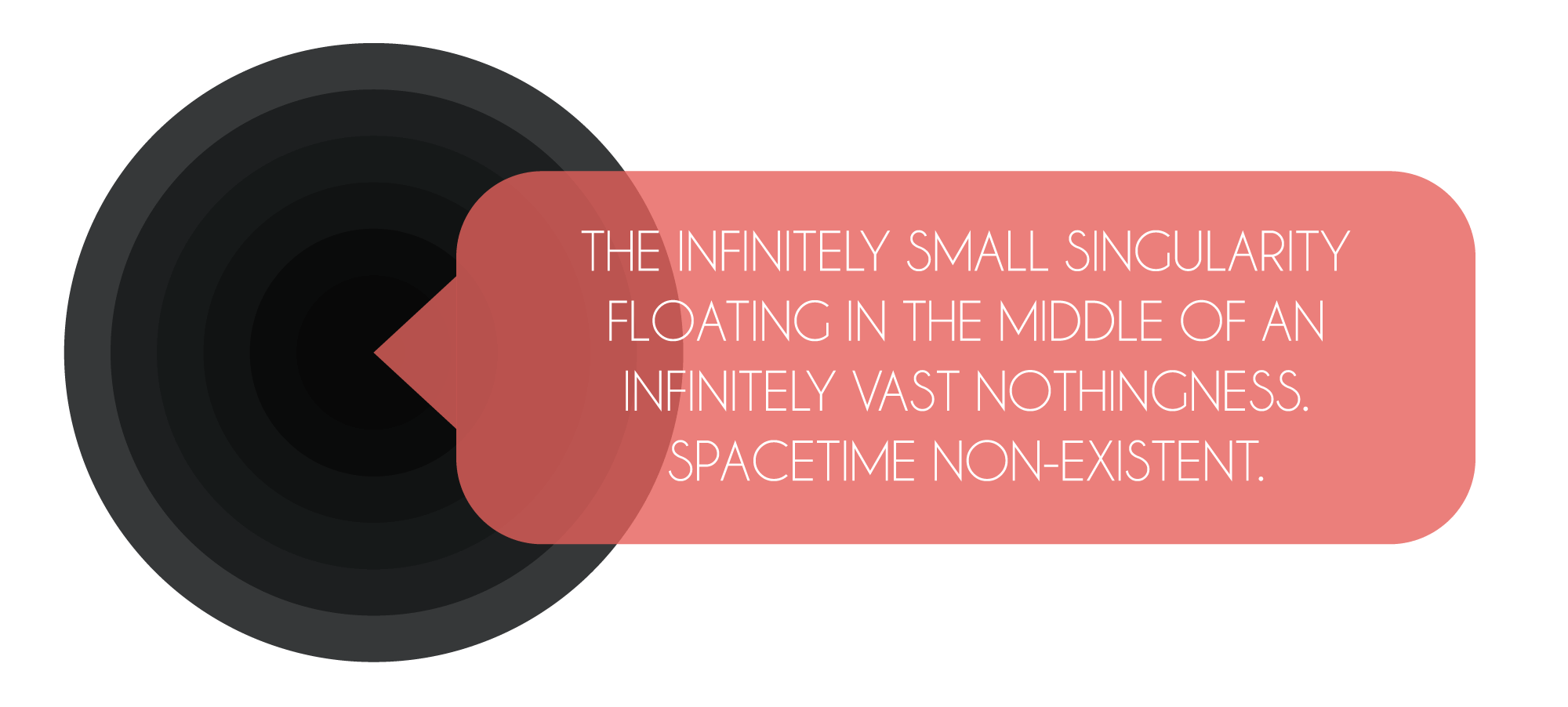
Back to our singularity. It would be fitting to say that it was an exceptionally peculiar object. We do not get to see such objects every day – never, in fact (unless we find ourselves in the centre of a black hole, which would probably not be an unduly pleasant experience – we are going to look into that in one of the following chapters).
Why was the initial singularity so peculiar? First of all, it may have been infinitely small, which is more than remarkable. On top of that, it was infinitely dense and infinitely hot, which makes good sense given that the entire universe had to fit into its heart. Exactly – everything you see around you has once been squeezed into this ancient singularity, though in a somewhat different form.
Suddenly, something incredible happened. Something that may be considered the strangest and most mysterious event of all time. The singularity started rapidly expanding and created the entire unbelievably huge universe. This incident is known under the majestic name of the Big Bang. However, it is rather ironic that we are using this name. First of all, this expression was coined by a man named Fred Hoyle, who was a tremendous opponent of the Big Bang theory (he favoured the competing Steady State theory). He only used this expression to mock the theory. In addition, the term “Big Bang” is incredibly inaccurate – it is far from representing the event that actually occurred.
Let us focus on the word Bang first. This word seems to represent a grand explosion accompanied by stunning sound effects. Much to the dismay of action movie fans, the actual “Big Bang” could be considered the exact opposite of such an explosion. There is no sound in vacuum, so let us forget about amazing sound effects. But more importantly, the Big Bang was not an explosion at all – it was more like an inflation of space (incredibly fast inflation indeed).
A great parallel is the inflation of a balloon. Imagine that our universe is represented by the surface of a balloon. If we inflate it, the distance between any two points on its surface increases. This is what the Big Bang and the following expansion of space looked like – it started off as an infinitely small point in the form of a singularity, and ended up as the surface of a gigantic “space balloon”. (Except that the surface of a balloon is a two-dimensional space curved in a third dimension, while our own universe is most likely a three-dimensional space curved in a fourth dimension. However, our primitive three-dimensional brains are not capable of imagining fourth-dimensional space – that is why we are using a simple balloon analogy.)
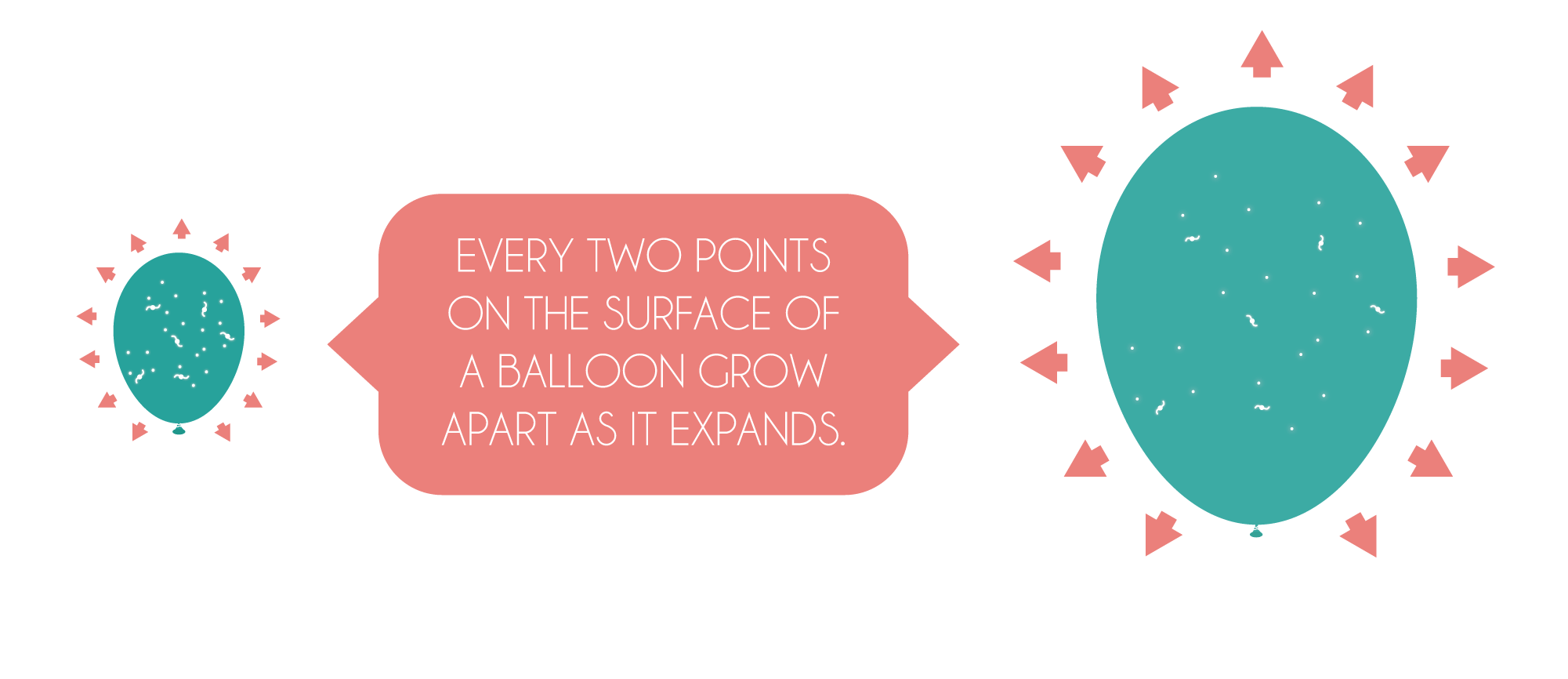
Now that we grasp the issues with the word Bang, let us focus our attention on the word Big. While the word Bang is utterly inaccurate, the word Big is correct. However, it is a tremendous understatement. The Big Bang was not only big, it was everywhere – every single tiny bit of space used to be condensed in an infinitely small singularity and underwent a whopping expansion. To augment our balloon metaphor, imagine that you are a two-dimensional ant inhabiting the surface of the expanding balloon. For such an ant, the surface of the balloon is the only thing in existence – the ant cannot escape it on its own. From its point of view, everything is inflating. For the sake of accuracy, it would be appropriate to replace the word Big with the word Huge – or even better, Omnipresent.
However, if we put these two accurate expressions together, we get something like the Omnipresent Inflation, which, let us admit, does not sound nearly as good as the Big Bang. We will therefore stick with the usual term, now with the knowledge of its imperfections.
One of the greatest questions is when exactly the Big Bang occurred. This immensely interesting question has been subject to discussion for decades. Today, it is assumed that the singularity started expanding astounding 13.8 billion years ago. You do not even have to try to understand how long ago it was – for the human brain, it is practically impossible. But we can at least try to present this information in a different way – imagine that you put marbles one centimetre (0.4 inches) in diameter next to each other into a single row. If each marble represented one year of the universe’s existence, your row of marbles would go around the entire Earth. Three times. And if you by any chance wanted to live through the whole age of the universe, you would have to live incredible 200 million average human lives. For comparison, the Earth is about 4.6 billion years old – about 67 million lives.
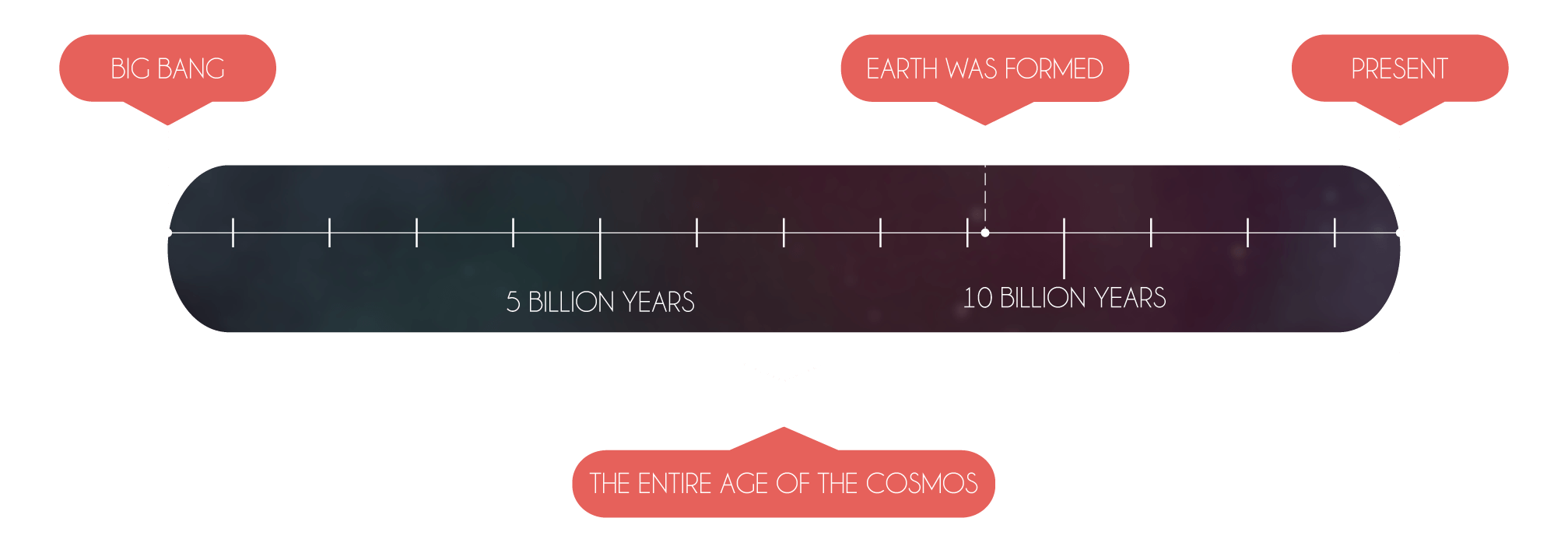
Let us come back to the very beginning now. No one really knows why the Big Bang occurred, but we do know quite well how exactly this process took place. 13.8 billion years ago, the singularity, containing the entire energy of the cosmos, started rapidly expanding. This moment is considered to be the beginning of space-time as well as the universe itself.
But what happened before the Big Bang? This seemingly interesting question is actually pointless. Time is inherently woven into the structure of our universe. Before the Big Bang, it simply did not exist. Asking this question is like wondering about what is located north of the North Pole.
As you have surely already understood, most things about the singularity are extreme and unimaginable. And the super-rapid expansion of the early cosmos right after the Big Bang is surely no exception. Trying to imagine any of the numbers bellow is simply impossible.
About a billionth of a billionth of a billionth of a second after the Big Bang, the universe entered a monumental state known as the cosmic inflation. It should be noted that the temperature of the cosmos at that time was respectable 100 million million million million degrees Celsius! During the cosmic inflation, the universe increased its size in an unimaginable manner. From the initial size of less than a billionth the size of a proton, it inflated to a comparatively huge sphere 1 centimetre (0.4 inches) in diameter. But even more remarkable is the fact that it has managed to alter its size like this in a mere fraction of a second – the cosmic inflation started about 10-37 seconds after the Big Bang and ended somewhere between 10-35 seconds a 10-32 seconds after the birth of the cosmos.
And in a blink of an eye, the cosmic inflation was gone forever. Space-time continued on its expanding journey (in fact, it continues expanding to this day), but much more slowly. At the end of the inflation, the temperature of the universe was about a 100 thousand times smaller than at its beginning. It is mesmerizing that in a moment much shorter than anyone can even imagine, the universe changed so dramatically. But what is most important – once the cosmic inflation was over, the cosmos already contained a crucial component which makes our very existence possible – matter.
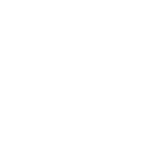
![]() |
![]() |
![]() |
PREVIOUS |
SHARE |
NEXT |
THE EVOLUTION OF MATTER
Every single object you see around you is made up of various atoms – tiny grains of matter. The human body, for instance, is composed predominantly from oxygen, carbon, hydrogen and nitrogen atoms. However, all atoms consist of even smaller grains – protons, neutrons and electrons. The number of protons inside an atom determines what kind of atom (or chemical element) we are dealing with. If you see an atom with only one proton in its core, it is surely hydrogen. If you encounter an atom with two protons, you are without any doubt dealing with a helium atom. Six protons? Carbon. Eight? Oxygen. We could go like this all the way to the number 92 – the number of protons in uranium, which is the heaviest natural element of the universe. 2
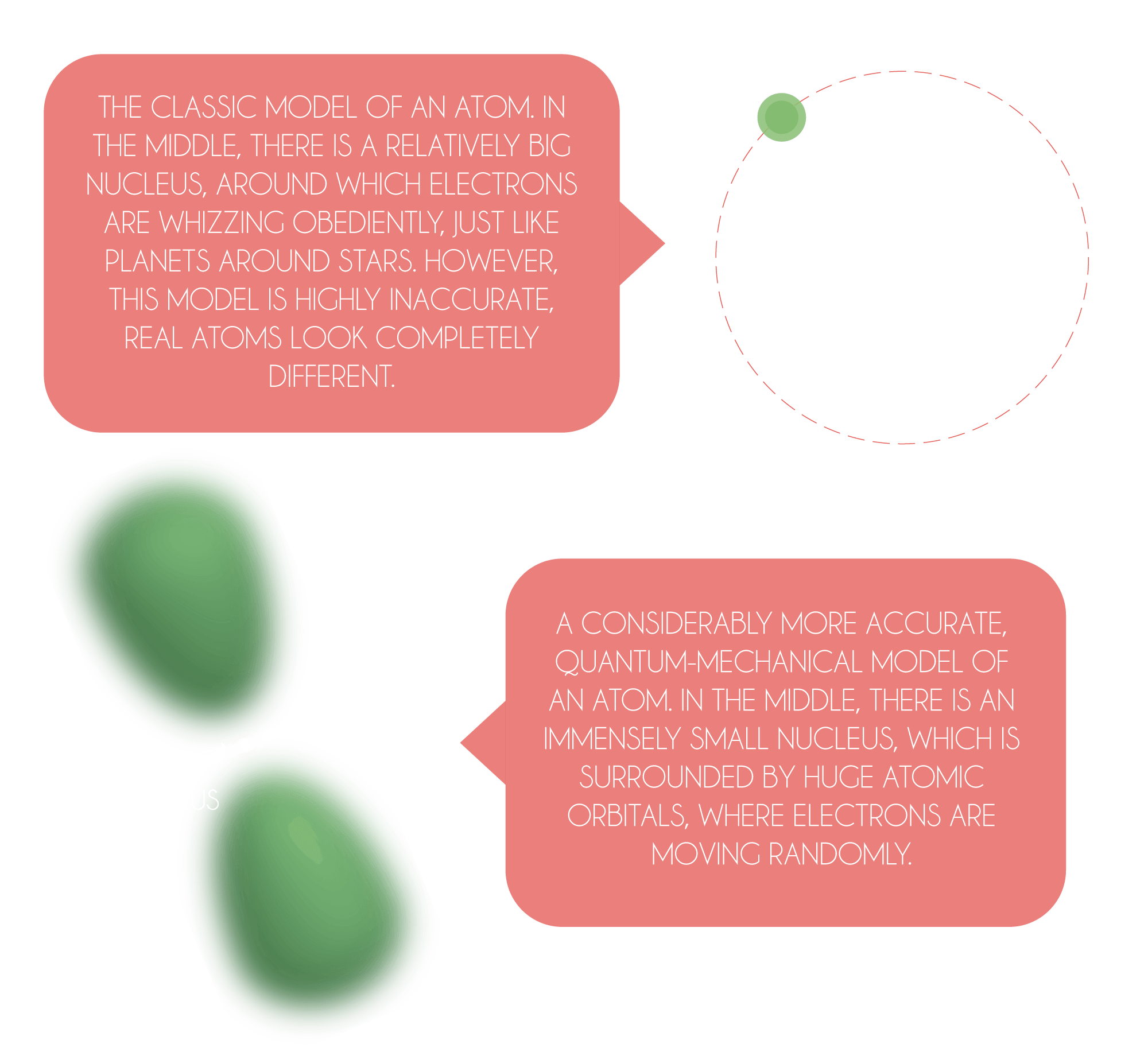
Your own atoms make up almost your entire mass. Every time you step on a scale, you measure the collective mass of all tangible subatomic particles that inhabit your body. But there is one important question – where do all of these particles come from?
To comprehend the sudden appearance of matter in the early cosmos, we first need to focus on the most famous physical equation on the planet, whose author is a world-renowned physicist Albert Einstein. E = mc2. Energy is equal to mass times the speed of light squared. Nice, you might say, but what exactly does it mean? Simply said, this brief equation daringly states that energy and mass are nearly the same thing. The only “converter” between the two quantities is the speed of light squared.
Take any object and multiply its mass by approximately 90 million billion – the value of the speed of light in meters per second squared. If you do that, you discover the immense amount of energy hidden inside the object. And you can do it in reverse too – if you take an arbitrary amount of energy and divide it by 90 million billion, you get its mass.
Exactly. Every form of energy weights something. A hot cup of tea is heavier than a cold one, as it contains more heat energy. However, you do not need to experiment and try to verify this fact by carefully weighing various tea cups at different temperatures – that is unless you live in a distant future where humanity is so technologically advanced that it can manufacture a hugely impressive scale which is able to detect differences of about a millionth of a millionth of a gram. Heat energy is far less concentrated than the energy we can find in matter. You would need to heat your cup to millions of degrees for a perceptible difference to appear.
The previous paragraphs could be summarised into one sentence – energy and matter are very closely related. So related, in fact, that you can create one out of the other. How? Well, if we consider the fact that each tiny bit of matter contains an enormous amount of concentrated energy, it would be logical to focus an unimaginable volume of energy to a single spot and hope that all of this energy would somehow “unite” and create a tangible particle. However, it is incredibly difficult to achieve that in today’s universe.
But if we consider how much condensed energy the early universe contained during the cosmic inflation (its temperature reached impressive billion billion degrees Celsius), we get stunning conditions for the creation of matter. The energy of the early cosmos was simply so concentrated that tangible particles started spontaneously forming.
The formation of matter definitely did not take long though – all of it was created during the unbelievably quick cosmic inflation. At its end, the universe contained nearly all the matter you can see around you. Every single one of billions of stars and galaxies consists of the same matter that was created just a fraction of a second after the Big Bing, when the universe was about the size of a grape.
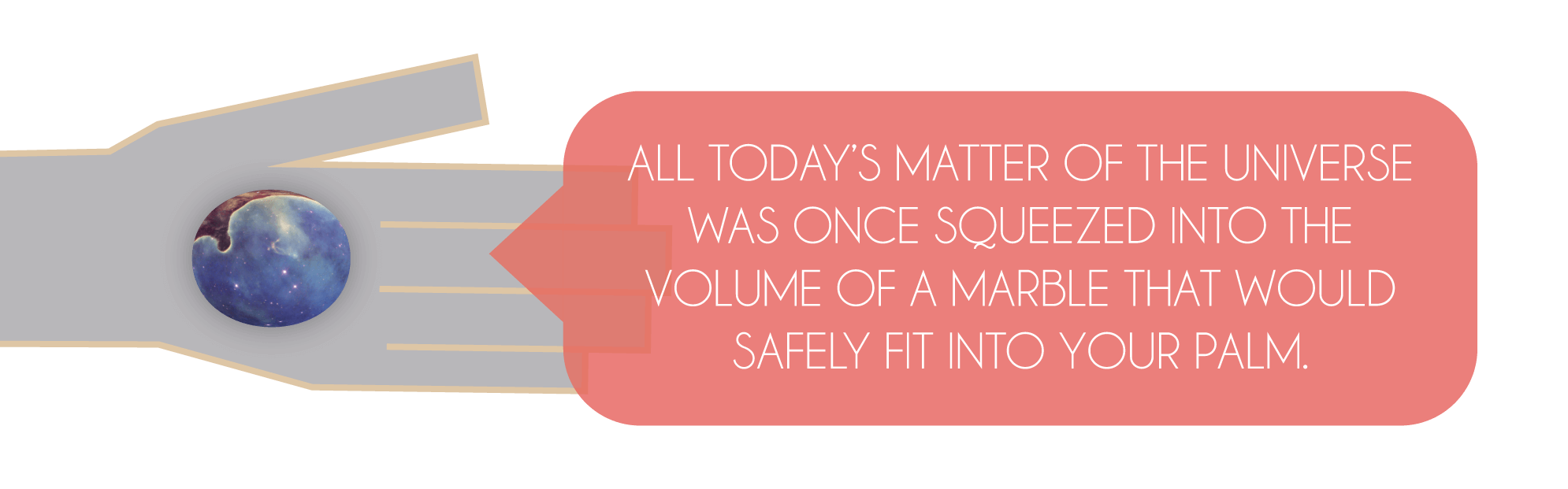
Back then, however, matter was far from forming atoms. For those, we have to wait several hundred thousand years. At that time, all matter was represented by the simplest of particles called quarks and leptons.
But there is a catch – the formation of matter is not that simple. There is a rule that with each particle of matter, its counterpart in the form of antimatter has to be created. Antimatter is just like normal matter, except that some of its properties are opposite – electric charge, colour or flavour. (The last two properties have obviously nothing to do with “our classical” flavour and colour – elementary particles cannot actually have any colour, since they are much smaller than the wavelength of visible light, not to mention flavour. They are just names physicists have given to various types of charges.) 3
But what is more interesting – matter and antimatter cannot stand each other. If they come into contact, both of them are destroyed in a violent explosion (this process is called annihilation) and all of their energy is transformed into photons – the particles of light.
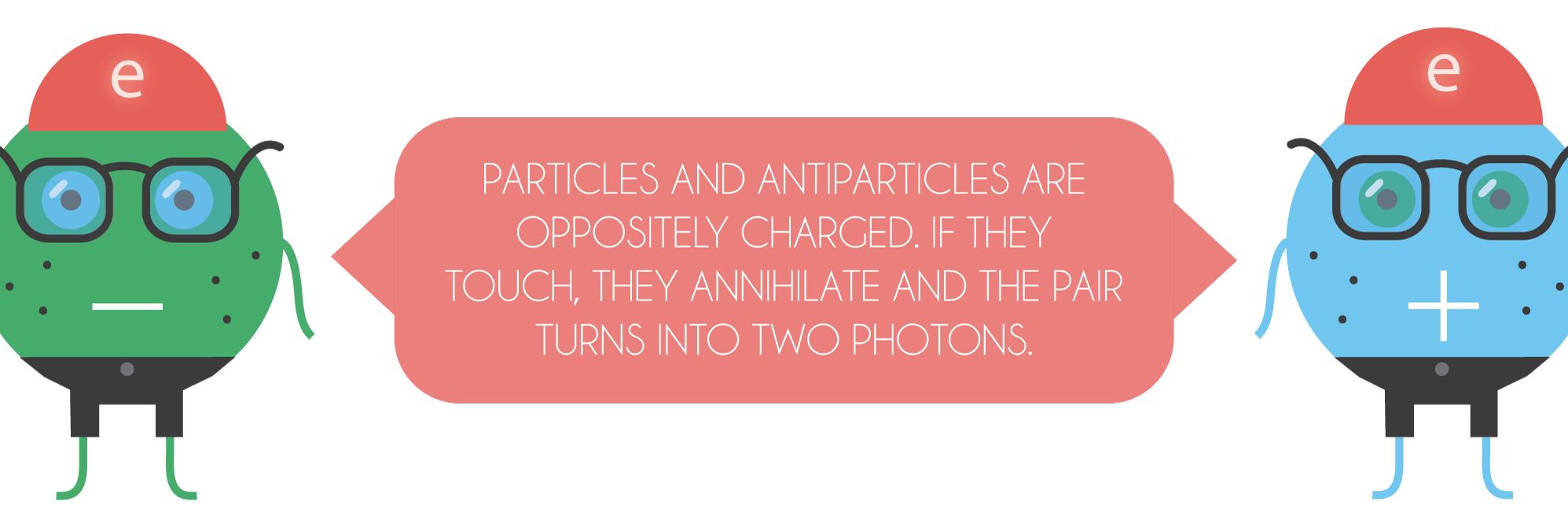
Let us go back to the creation of matter in the early cosmos. It follows from the previous paragraphs that all the matter which was produced just a moment after the Big Bang had to be accompanied by the same amount of antimatter – each tangible particle was created along with its antiparticle. And since the universe was so incredibly small back then, the contact of particles and antiparticles was simply inevitable. Most of the newly created tangible particles crashed into an antiparticle and perished just a moment after their birth.
But there is one significant question. Why is there still matter in the universe today? By the laws of physics, the exact same amount of matter and antimatter should have been created. Theoretically, it follows that mutual destruction of all matter and antimatter should have occurred in the young universe. But that did not happen – otherwise we would not be here.
Nobody knows why, but it seems that for every several million antiparticles, one extra particle was created. Each of these surplus particles avoided annihilation and formed all matter we can see in today’s universe. It is staggering when we realize that the early universe not only contained nearly all matter it does today, it contained much more of it. And all of that was squeezed into a volume that would fit into a human palm.
However, the energy released in matter-antimatter collisions did not disappear. It was transformed into photons of high-frequency radiation. These photons then kept on roaming the newly created universe, which was packed with charged tangible particles. These particles prevented the photons from moving freely. It took 380 000 years before the universe became transparent due to the formation of atoms and photons were finally able to travel unimpeded. Many of these photons keep on cruising the universe to this very day and constitute the cosmic microwave background – living evidence of the Big Bang.
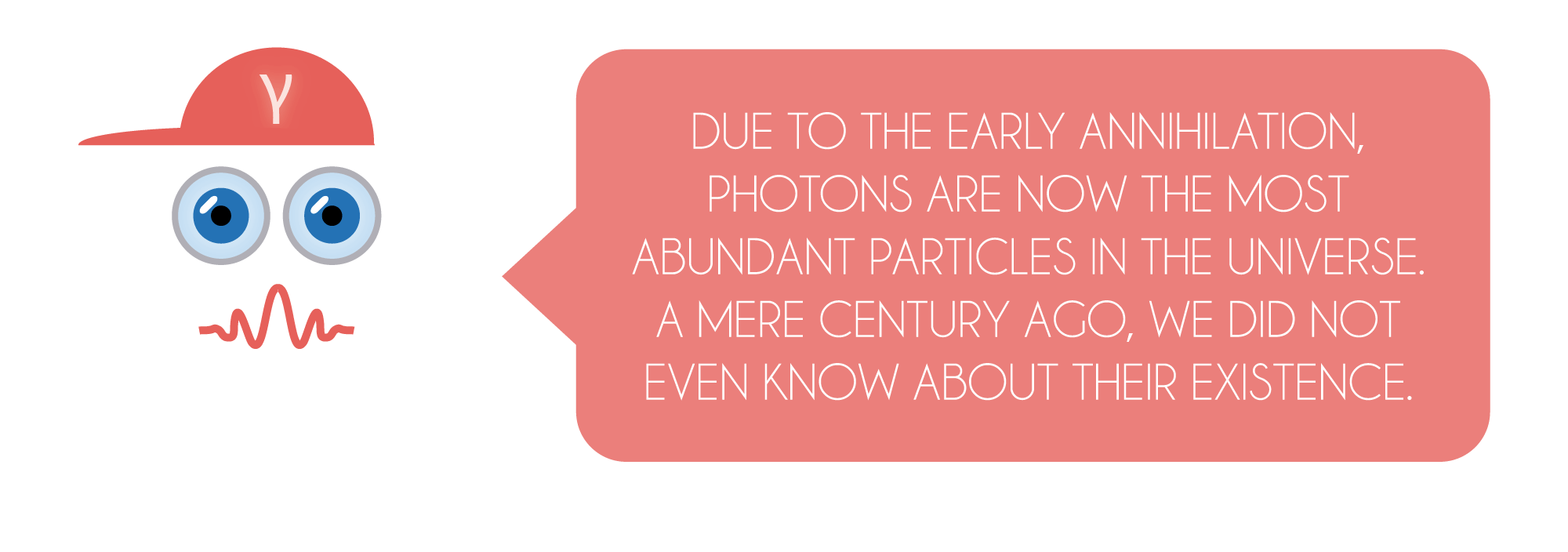
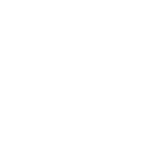
![]() |
![]() |
![]() |
PREVIOUS |
SHARE |
NEXT |
ATOMS, STARS AND THE REIGN OF GRAVITY
Once the first violent fraction of a second was over, the evolution of the universe slowed. A lot. The key expressions for the following development of the cosmos are cooling, expansion and the synthesis of simpler structures to create more complicated ones. About a millionth of a second after the Big Bang, the temperature of the universe decreased to such an extent that the simplest particles started joining to create more complicated particles – the first protons and neutrons came into existence. A few minutes after, these particles started clumping together and the first atomic nuclei saw the light of day. This process is called the nuclear fusion.
The temperature of the cosmos was approximately one billion degrees Celsius back then – still a breath-taking value but ridiculously small compared to the prior values. Just 20 minutes after the Big Bang, the temperature of the universe was no longer high enough to sustain nuclear fusion. The creation of new elements ceased for several million years – until the first stars initiated it again.
When the fusion stopped, three quarters of all matter in the universe formed hydrogen nuclei (the lightest element), the last quarter made up helium nuclei (the second lightest element). However, it took another 380 000 years before electrons bound to them, which flooded the cosmos with the first atoms.
380 000 years after the Big Bang, a new epoch of the universe began. Photons could finally move freely through space-time due to the creation of atoms. But what is more, a seemingly innocent force that was present almost from the very beginning slowly started to gain power – gravity. One of the following chapters is dedicated to this fascinating interaction, for now you only need to know one thing – every single object in the universe is attracted to every single other object, while the amplitude of the force with which they attract is proportional to the square of the distance between the two objects. What does it mean? Simply said, if two objects are one meter apart, the gravitational force between them is four times as great as if they were two meters apart.
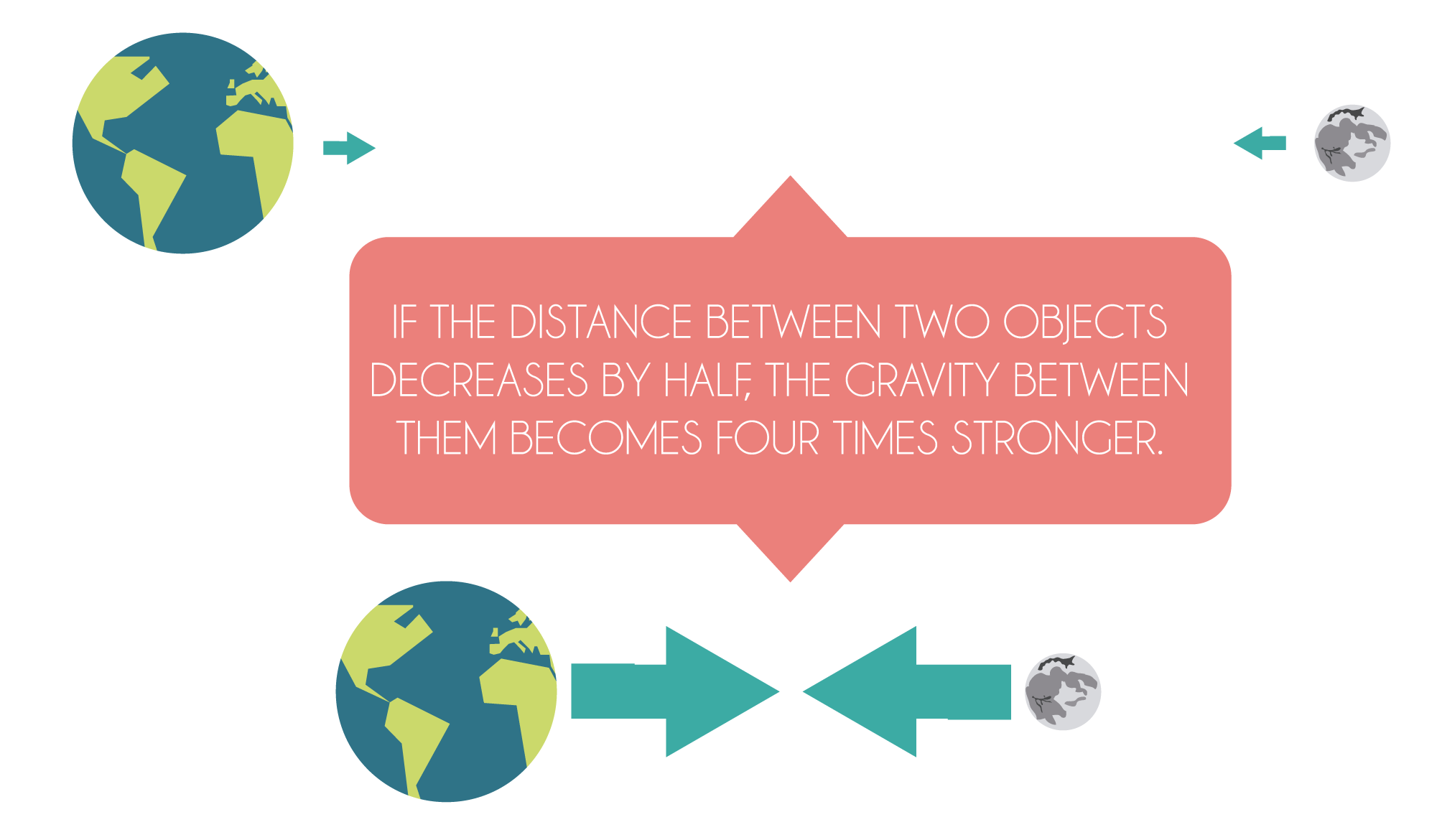
The gravitational force, even though it is the weakest of the four fundamental interactions (again, you will have to wait for the following chapter), has become the unquestionable dominant force of the universe. Right after the Big Bang, tiny disproportions in the distribution of matter were produced due to vacuum quantum fluctuations. Imagine spilling a handful of sugar on a paper. It is hugely unlikely that each section of the paper would contain the same number of sugar grains. On the contrary – some spots would contain large clusters of sugar, whereas others would hold no sugar at all. And something similar happened to the early cosmos – some sections of space-time simply contained more energy than other sections.
In places with a higher concentration of energy, more elementary particles, more atomic nuclei and eventually more atoms were created. This was crucial for the following development of the cosmos. Were it not for the early fluctuations causing disproportions in energy density, each bit of the universe would contain an identical amount of matter and gravity would never be able to show itself.
It is as if you tried to move a cube but you would keep pushing all faces with exactly the same force – the cube would stay in place no matter how large the force would be. However, if you applied just a little more force to one of the faces, the cube would start moving in the direction of the force. To some extent, this is what happened in the 380 000 years old universe. The parts of the cosmos with a higher concentration of matter gravitationally affected each other more and began happily attracting – the first nebulae saw the light of day.
Then, these nebulae were becoming denser and denser due to gravity and the temperature in their cores was gradually increasing. After several hundred million years, the temperature in their hearts was so high that nuclear fusion was ignited – the first generation of stars was born. These stars then went on to clump together into enormous formations called galaxies, which exist to this day and often contain up to billions of astral residents.
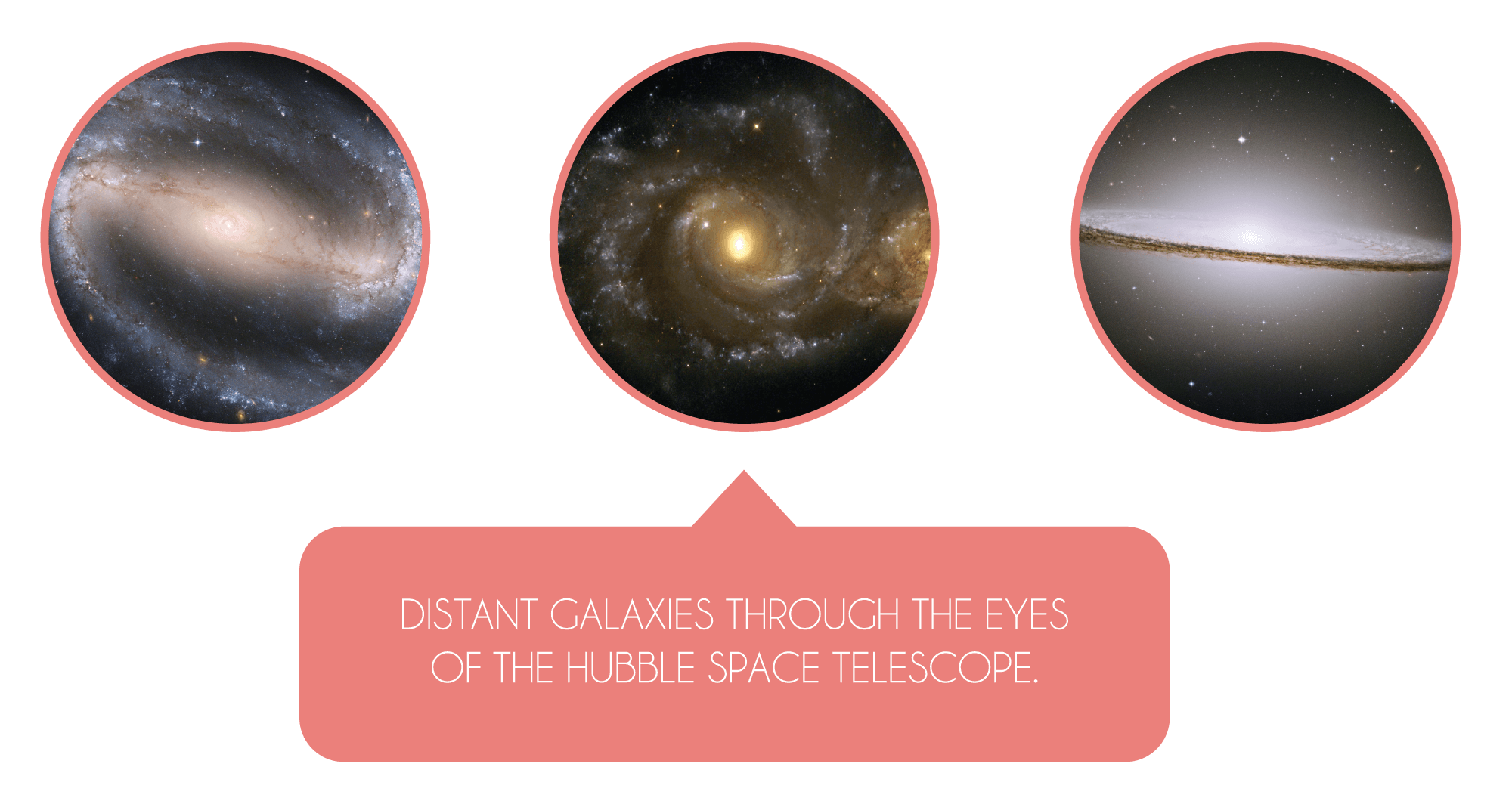
All that time, however, stars were doing something immensely important – they transformed simpler elements like hydrogen and helium into more complicated ones. The universe beheld elements like carbon, oxygen and iron for the first time. But every star has to die eventually. The early stars were usually gigantic and ended their lives in massive explosions, during which they ejected an enormous amount of material into the surrounding space.
The material then went on to create the next generation of nebulae and the entire process repeated – the nebulae formed new stars which in turn built more and more complicated elements. These were once again expelled into the adjacent vacuum. However, some of the elements started forming new structures, which had never existed before, called planets – smaller cosmic objects in which nuclear fusion is not ignited. Planets usually revolve around a parent star. Such a star was essential for the early planets, since it supplied them with necessary energy, which allowed various chemical reactions to occur. These reactions then enabled the formation of the first amino acids. Then, after many years of effort, at least one of the planets created the most complicated known entity in the whole universe – life.
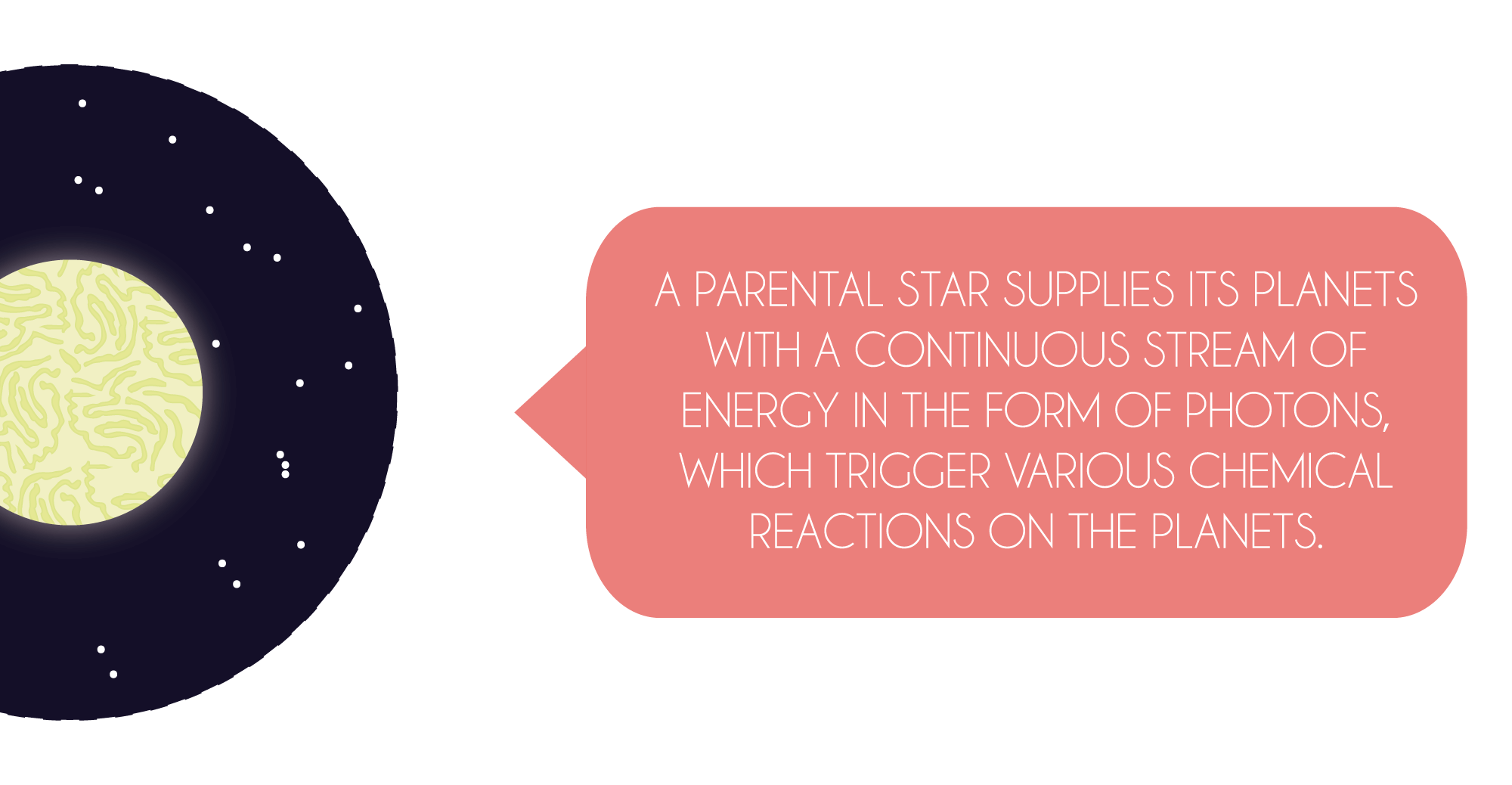
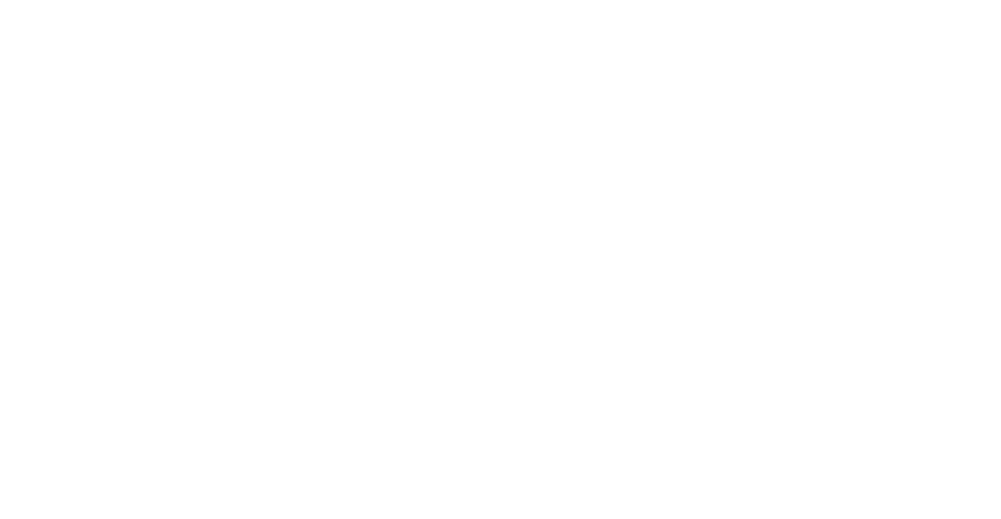
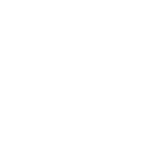
![]() |
![]() |
![]() |
PREVIOUS |
SHARE |
NEXT |