INTRODUCTION
People are quite good at estimating various things. To demonstrate what I mean, try answering the four following questions. How long does it take an average person to walk half a kilometre? Approximately how many eggs fit into small box? How much taller is an adult person compared to a six-year-old? What is the average difference in temperature between summer and winter? It is very likely that your answers to the questions above were to some extent correct.
Everybody knows that an ordinary human walks a distance of a few hundred meters in a couple of minutes, that about five to ten eggs fit into a small box, that a child is about two or three times smaller than an adult, and that the difference between summer and winter temperatures is several dozen degrees at most. No reasonable person would tell you that they can walk half a kilometre in about a tenth of a second, that about a billion eggs fit into a box, that a twenty-year-old is about a hundred thousand times larger than a child or that the temperatures in summer can be up to a million times greater than those in winter. Our brains are excellent at estimating these things, since we encounter them on a daily basis.
But once we get over the border of the ordinary world, our perspective suddenly vanishes. How many atoms fit into the dot of the question mark at the end of this sentence? How much farther from Earth is the Sun compared to the Moon? What is the difference in temperature between the Sun’s core and its surface? If you have not happened to learn the answer to one these questions, you might not be able to respond. And if you have at least tried, it is very likely that your answer is far off from reality. Our brains do not encounter atoms or stars on a daily basis, which makes answering these questions immensely difficult for them. The aim of this chapter is therefore to bring a little bit of perspective into the mysterious world of the cosmos.
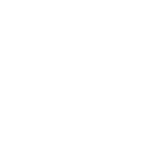
![]() |
NEXT |
ASTOUNDING COSMIC SIZES
Our world is much smaller but also much bigger than most of us think. On one hand, there is an incredibly minuscule world of atoms and molecules, on the other hand, we can see a gigantic world of stellar giants. Let us start with the first group – the smallest objects of the cosmos.
What is the smallest thing in the universe? Nobody knows. Obvious candidates could be singularities in the hearts of black holes – anything smaller than these dimensionless points can logically not exist. However, there is one problem – nobody can prove that singularities are real. The existence of these infinitely dense points stems from Einstein’s theory of relativity. But general relativity, as I have already mentioned in the chapter about gravity, stops working at the subatomic level. It is therefore a little dubious to rely on the existence of this smallest thing in the universe, just because it is predicted by a theory that is known for its imperfections at the microscopic level. That is why some scientists deny the existence of singularities and firmly believe that the true dimensions of the mysterious objects in the centers of black holes will be revealed by the theory of everything. Let us therefore move to the second smallest “thing” in the universe – the Planck length.
Max Planck was a German physicists and the father of quantum mechanics. He gave us one of the most important physical constants – the Planck constant. Using this constant, it is possible to calculate the Planck length – the theoretical smallest distance in the universe. Below the level of the Planck length, the whole concept of distance simply stops making sense. How long is the Planck length then? In the order of ten billionths of a billionth of a billionth of a billionth of a meter. This length, however, carries the same problem as singularities – somebody has yet to prove that the concept of the Planck length really is embedded within our universe. Until that happens, it is merely an interesting concept of quantum mechanics.
Let us therefore move to the next candidate – elementary particles. An elementary particle is any particle which, according to the Standard Model (a theory that beautifully categorises all known particles), does not have any structure. These particles include for instance electrons or quarks, from which protons and neutrons are made. And since they have no structure, they are considered to be point-like – similarly to singularities.
But it gets a little more complicated – two problems arise. Firstly, nobody has yet proven that elementary particles are indeed elementary and therefore have no structure. It may be possible that electrons and quarks are made up of even smaller and simpler particles. 1 And the second problem comes from quantum mechanics. According to this peculiar theory, all particles are also waves. Imagine an ocean wave that turns into a point any time you wish to observe it – this is how electrons as well as other particles sometimes behave. It is therefore treacherous to deal with the dimensions of elementary particles – sometimes they appear to be dimensionless points, other times they behave like relatively large waves.
If you are getting tired of objects whose size is a bit of a mystery to us, I have amazing news for you in the form of an atom. On the atomic level, quantum mechanics loses its power and this whole wave-particle duality is suddenly gone. The radius of a hydrogen nucleus (the lightest element of the cosmos) is about ten billionths of a meter. If you take one millimetre and split it into ten million parts, each of these parts will be the same size as one of these atoms. And the whole thing becomes even more interesting if we realise that a hydrogen nucleus by itself is another thousand times smaller than that.
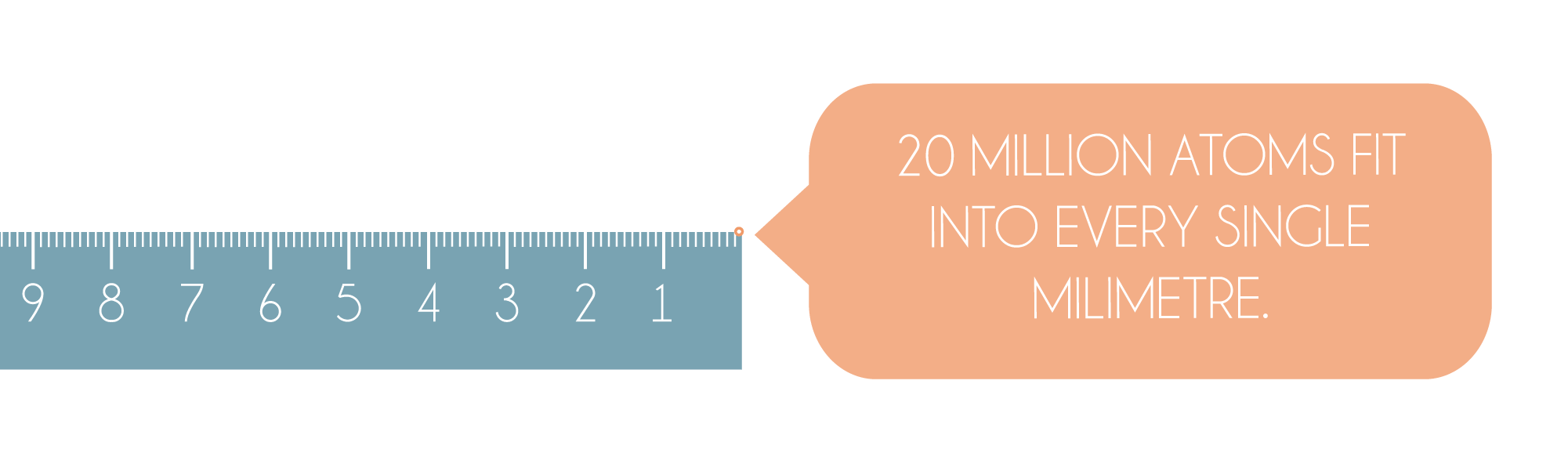
Imagine that we enlarge an entire hydrogen atom to the size of about ten centimetres. To preserve the ratio, we enlarge a marble that was originally ten centimetres in diameter by the same multiple. How large would such a marble be? It would turn into a gigantic sphere eight times bigger than the whole Earth. Here, we can beautifully observe how incredibly small atoms are in comparison to ordinary objects.
To take the demonstration of the tininess of atoms a bit further, take a pencil and a piece of paper. Now, draw a small dot on the paper. If you have done so, you have stained your paper with billions of carbon atoms. However, this number is ridiculously small if we compare it to the number of atoms in an average-sized human body – staggering seven billion billion billion. 2
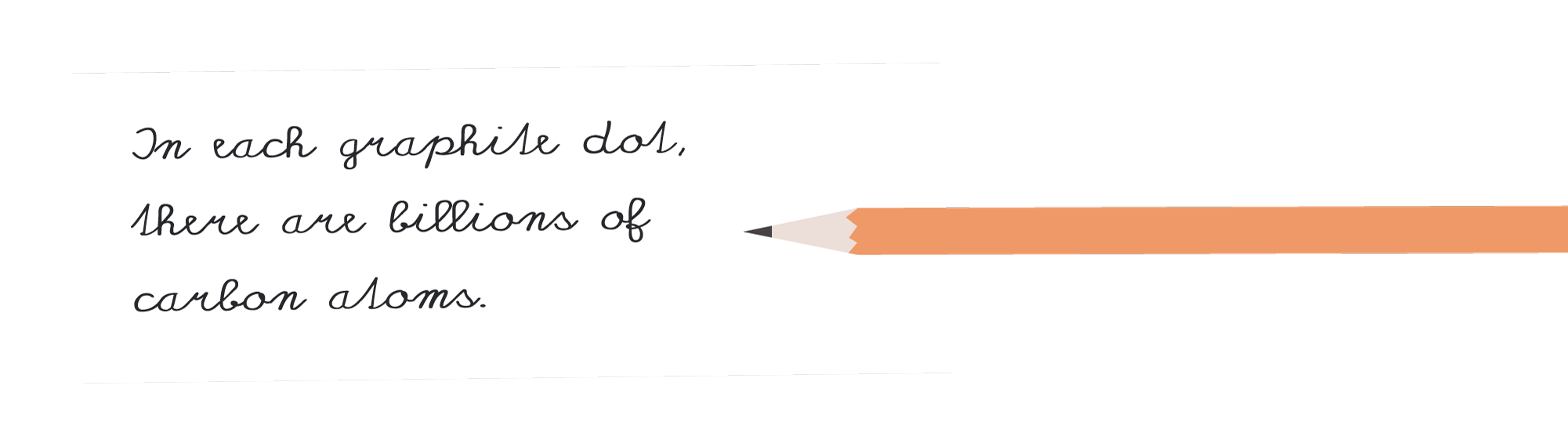
Even if we consider the bodies of the smallest and simplest existing organisms, bacteria, we still get a huge number of atoms – billions. Bacteria are one of the first organisms that appeared here on Earth 3.8 billion years ago. Their body is made up of just one prokaryotic cell. The size of such a cell is about a millionth of a meter. That might seem like an incredibly small number, but cells are truly gigantic compared to atoms.
Our next stop on the scale of sizes are eukaryotic cells – the elementary buildings blocks of many organisms, including humans. They are usually about ten times bigger than bacteria cells, but their size differs a lot. The smallest of them can be as small as prokaryotic cells. The largest can measure up to a few meters – the cells of the nervous system inside large animals. The human body contains tens of millions of millions of eukaryotic cells and none of them are able to exist by themselves. However, together they can do wonderful things – every complicated organism is in its essence just a collection of cells. 3
Now we can move from the world of indescribably small to the world of incredibly big. The planet you are currently inhabiting is a huge sphere almost 13 thousand kilometres in diameter. If you were to go around the entire circumference of the Earth (which is not a very good idea since almost three quarters of the Earth’s surface are made up of ocean, whose average depth is four kilometres), it would take you nearly a year. 4
If we explore the immediate vicinity of the Earth, we can see a single natural satellite, created about 4.5 billion years ago, when our planet was hit by a mysterious space object about half the size of Earth. This gigantic collision ejected a tremendous amount of material to Earth’s orbit, which then began to join into a single bulky ball revolving around our planet – the Moon.
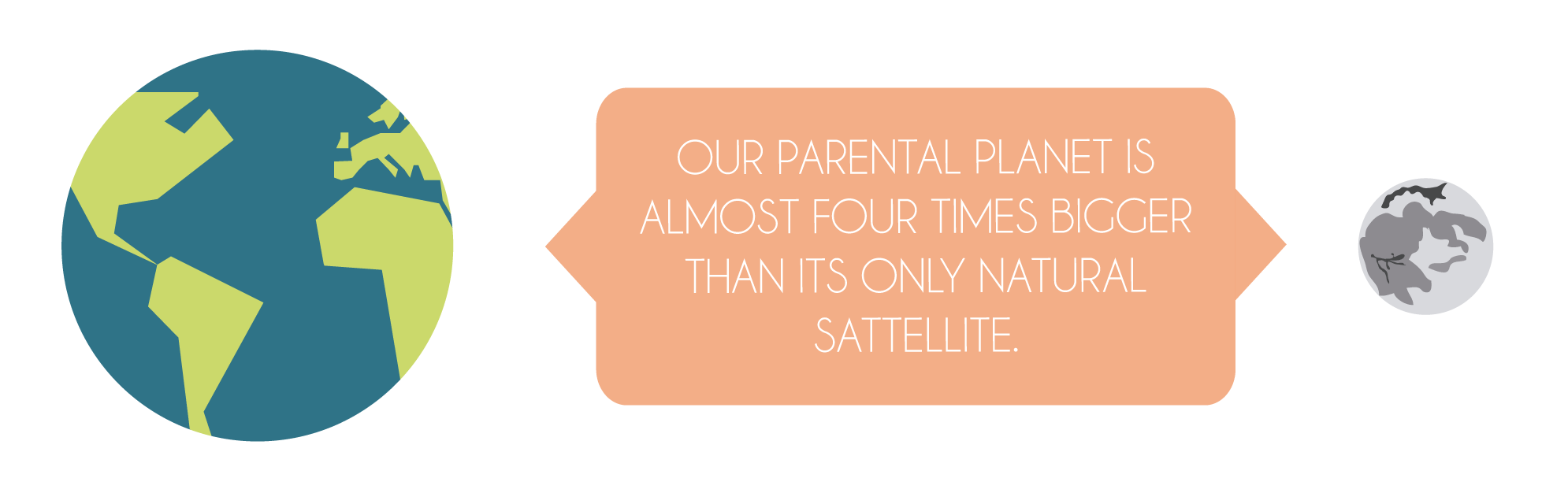
The Moon’s radius is about 3.7 times smaller than that of Earth. But it is not the only moon in our solar system – even other planets have their own satellites. For example, our planetary neighbour Mars has got two – Deimos and Phobos. However, both are significantly smaller than our Moon. But the true champion of moons is the largest planet of the Solar System – Jupiter. Today, we know of 67 Jupiter’s moons – a number that is very likely not final.
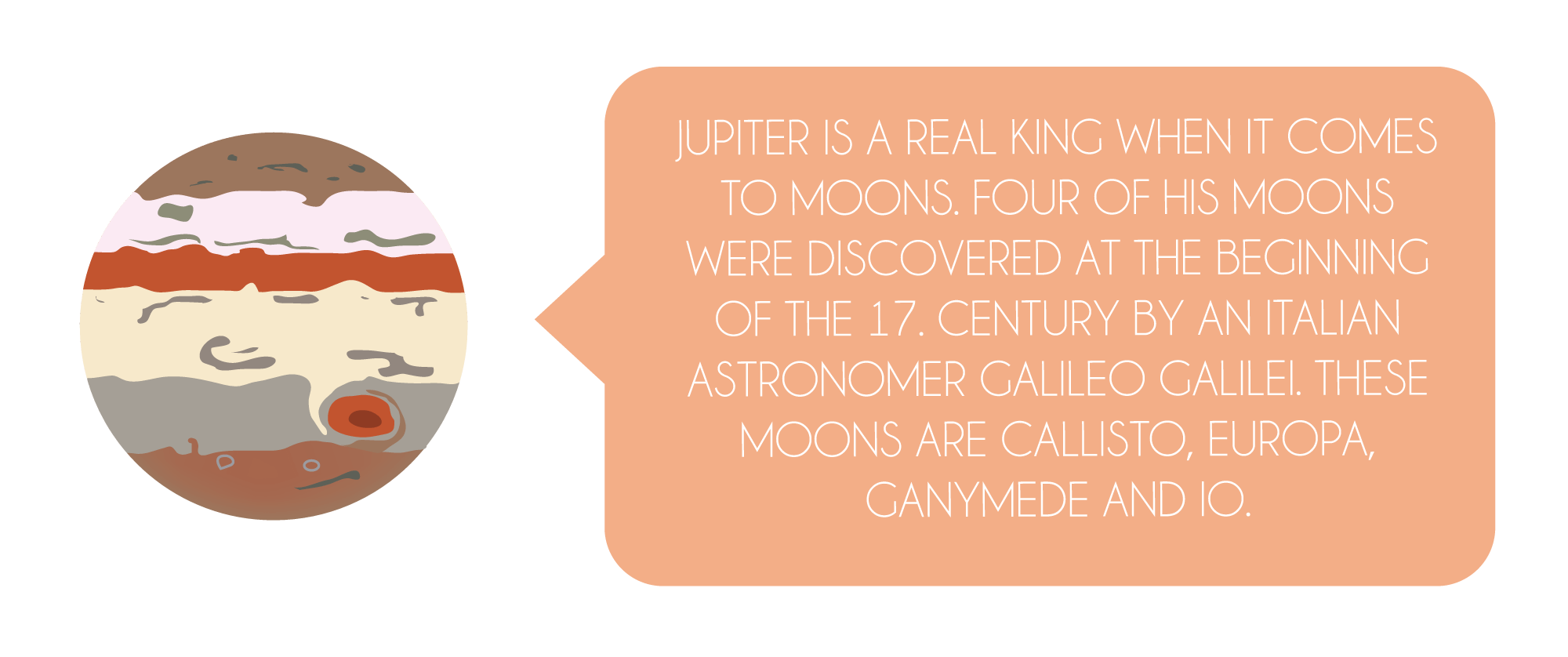
The biggest moon of the Solar System is Ganymede, with its five thousand kilometres in diameter. This moon is actually even bigger than the smallest planet of the Solar System – Mercury. The second smallest planet is Mars, followed by Venus, which is only slightly smaller than Earth. Together, these four objects constitute terrestrial planets. All of them have a solid surface and their size is not much different from that of our own blue planet.
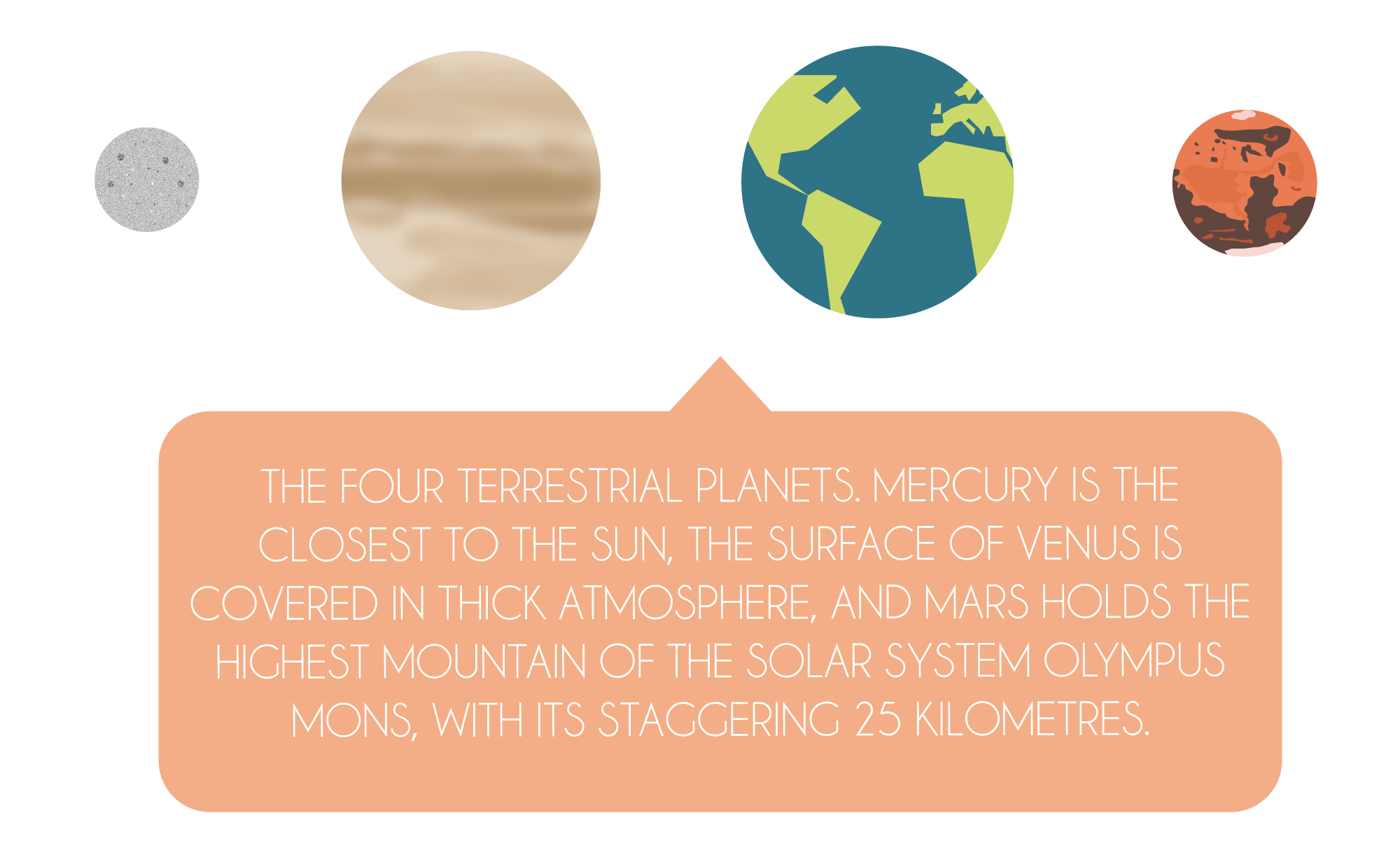
However, the remaining four planets of the Solar System (Jupiter, Saturn, Uranus and Neptune) are completely different. You would not find any solid surface on them – they are all gas giants. Except for a tiny solid nucleus, they are made up purely of gases. On top of that, they are much larger than the first quaternion of planets, as the name giants itself implies. Take Jupiter, for example, which is with its 140 thousand kilometres in diameter about eleven times larger than the Earth. You would have to travel a total of ten years to walk the distance equal to the circumference of Jupiter (even if you travelled by car at the speed of 100 kilometres per hour, this journey would take you more than half a year). But what is more, if you wanted to fill the entre volume of Jupiter with Earths, you would need staggering 1300 of them.
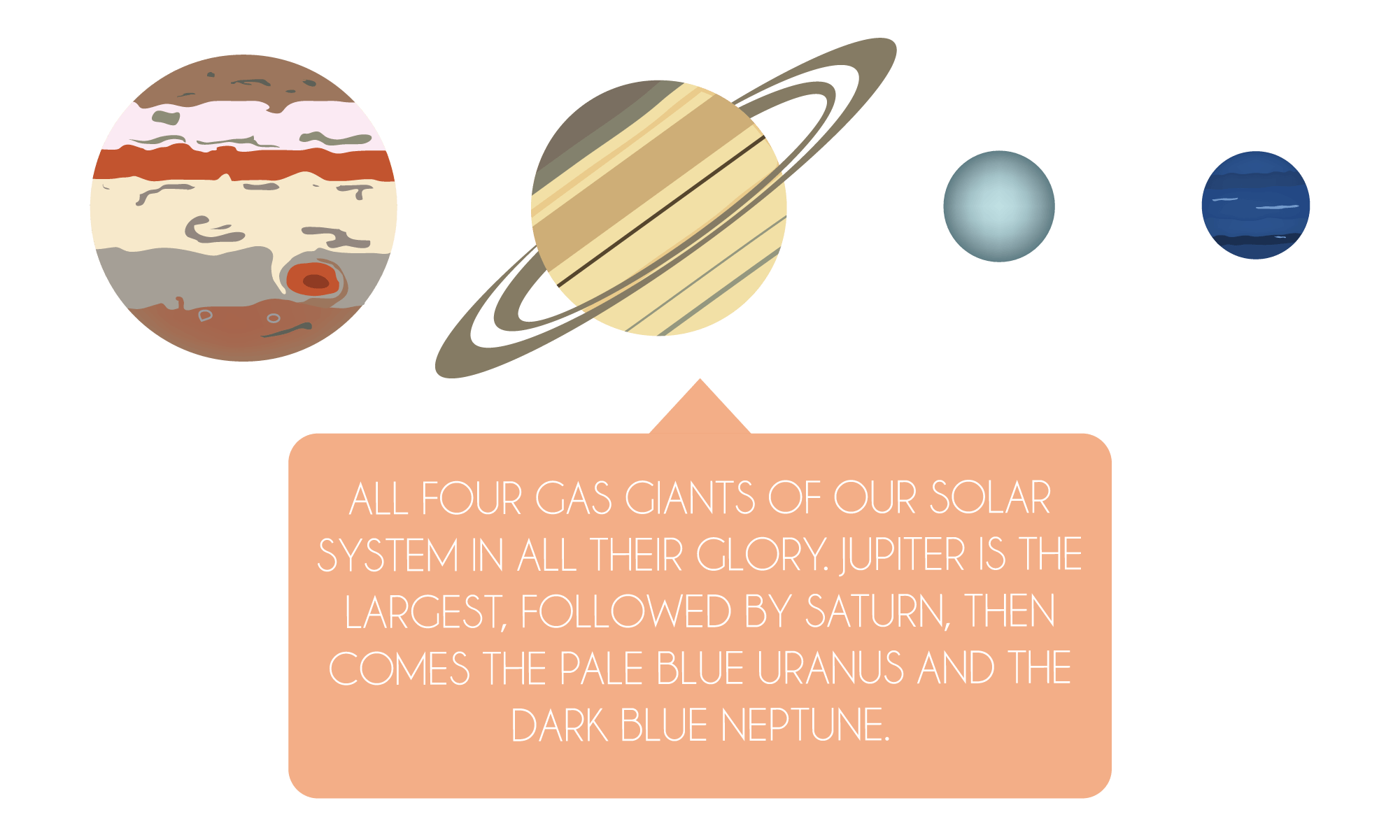
But even a giant like Jupiter is nothing compared to the king of our Solar System – the Sun itself. Its diameter is incredible 1.4 million kilometres, which is the equivalent of 110 Earths. If the Earth were a marble the size of a ping-pong ball, the Sun would be substituted by a huge ball 4.5 meters in diameter. It would take you about five years to drive around the entire circumference of the Sun (and half a year to fly around it in an airplane), and to equalize the Sun’s gigantic volume, you would need over one million Earths.
But what is the largest object of the universe? Unfortunately, nobody knows. But we have a few candidates. The largest star we know of is a red giant UY Scuti, which has a monstrous diameter of 2.4 billion kilometres – about 1700 Suns put side by side in a row. But again, I am not going to omit a little comparison with human vehicles – in a car, you would encircle this staggering star in incredible eight thousand years.
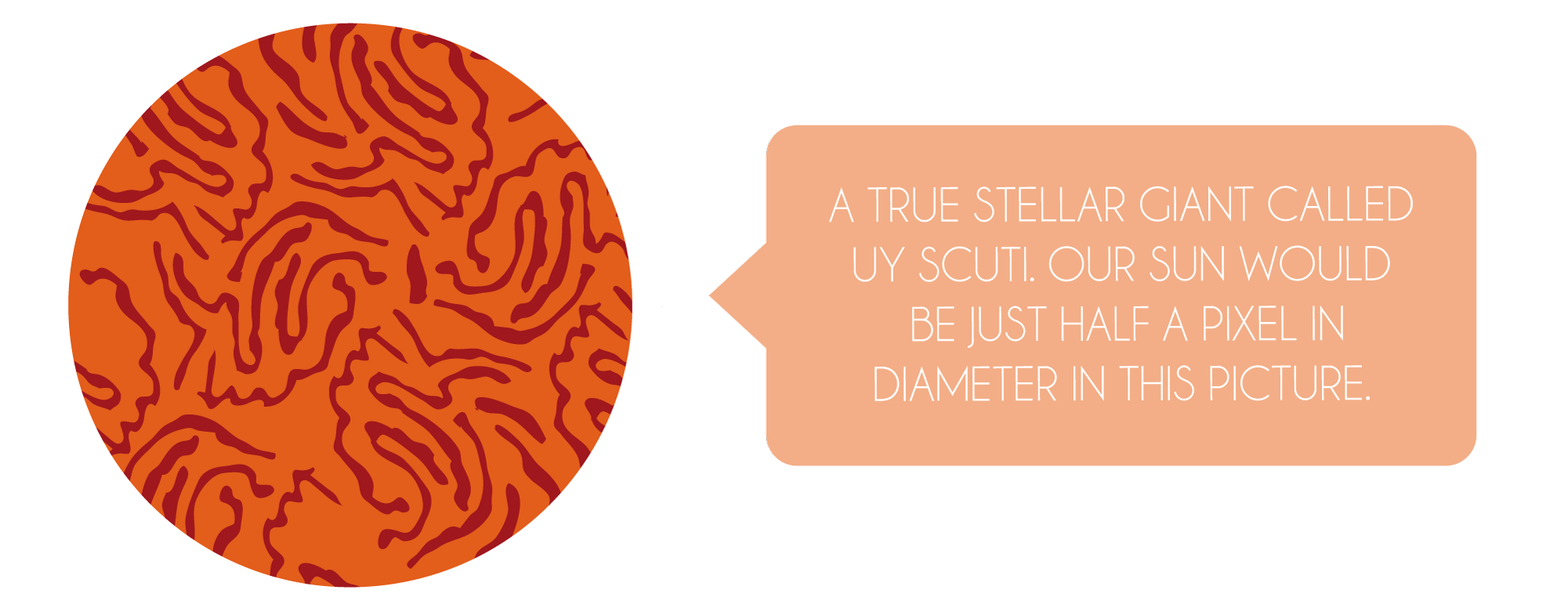
But the largest supermassive 5 black hole we know of is a lot bigger than that. Its diameter is over 90 billion kilometres. That is the equivalent of 600 distances from the Earth to the Sun! Even light moving at incredible 300 000 kilometres per second would need four days to get across the whole supermassive black hole.
The following objects on the cosmic scale of sizes are somewhat dubious and bring us to the following question: What could even be considered an object? If we accepted all the gigantic cosmic formations as objects, we would get an entirely new set of candidates. Our own galaxy is about 100 thousand light years across. But some galaxies are so big that it takes light a few million years to travel all the way through them.
But enough about sizes. Now, we can move to another foggy aspect of the cosmos – distances.
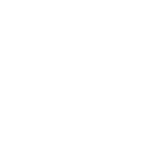
![]() |
![]() |
![]() |
PREVIOUS |
SHARE |
NEXT |
GIGANTIC COSMIC DISTANCES
Look at this picture of the Solar System. You may have encountered similar ones in various school text books. Huge Sun on the left, followed by planets, which are arranged by their distance from our parental star.
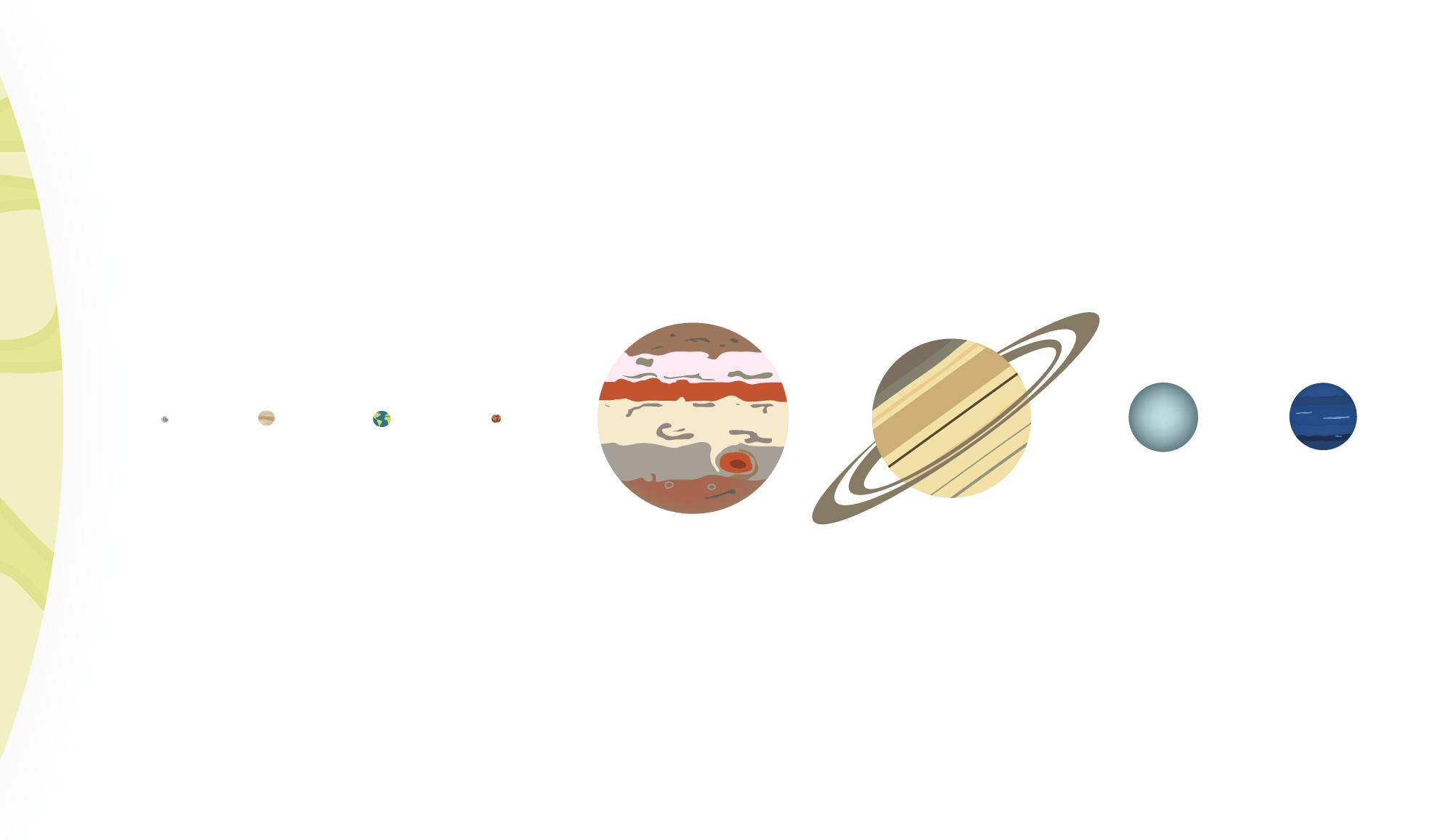
Unfortunately, this picture is far away from describing our Solar System in an accurate way. There is a huge problem, as you might have already guessed, with distances. A more accurate model of the Solar System would look something like this:
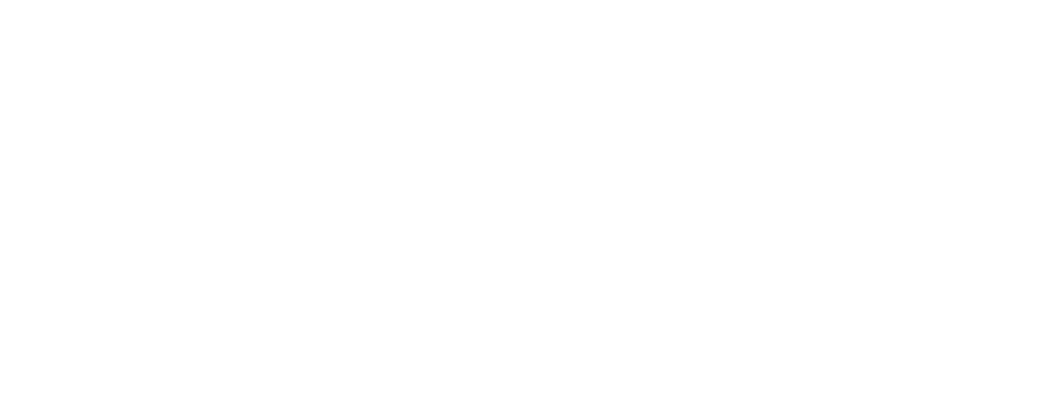
You cannot see anything? Exactly. Individual objects are so incredibly far apart that it is simply impossible to symbolise their distance while preserving a reasonable size of the planets. Even the Sun itself, by far the largest object of the Solar System, would have a diameter of less than just one pixel on the image above. It is as if you scaled down the distance between New York and London on an A4 sheet of paper and expected to see an accurately shown structure of both cities – you would be lucky if you saw to minuscule dots, each on the opposite side of the paper. Simply said, the distance between cosmic objects is much greater than the size of the objects themselves.
After all, we can demonstrate that on our own Moon. It may not seem like it, but the Moon is actually very far away from the Earth. If the Earth were the size of a football, the Moon would be a slightly diminished tennis ball 6.6 meters away from the football. An accurate depiction of the distance looks like this:

However, that is nothing in comparison to the staggering distances between stars in the galaxy. Proxima Centauri is a small red dwarf invisible to the naked eye. But it is a very special star for us – right after the Sun, it is closest to us. It is still incredibly far away though – about 40 million million kilometres. Its light has to travel for more than four years to reach us. And other stars of our galaxy are much further.
Sirius, the brightest star of the night sky, is approximately 8 light years away from us. 6 The light of Polaris (a star that is located right above the North Pole and thereby gives the illusion of a static star around which all the others revolve) takes more than 400 years to get to Earth. And if you were light and wished to travel to a star on the other side of the galaxy, you would have to get prepared for a very tedious and monotonous journey lasting a few thousand years.
However, we can only grasp the real vastness of space on the level of galaxies. Compared to the staggering distances between galaxies, all the distances I have mentioned up to this point are ridiculously minuscule. For example, the Andromeda galaxy, which is our closest spiral galaxy, is about 2.5 million light years away (24 million million million kilomteres). Some other galaxies of the Local Group (a galactic formation you are currently inhabiting) can be even further.
The entire Local Group is a part of an even larger galactic structure – the Local Supercluster. This entire formidable formation is over 110 million light years across.
All these numbers reveal two cruel facts about the universe. In the first place, it tells us how tiny we actually are. People used to think that the Earth is the centre of the entire universe. But then we discovered that the truth is a little different – the Earth is a miniature planet orbiting one of billions of stars of the Milky Way, which is just one of billions of galaxies of the whole observable universe. At the largest cosmic scales, we are simply nothing.
The second cruel fact is related to the space exploration. Who would not dream of an exciting trip to a different galaxy? The truth is, however, that it will take many years before we are even able to start exploring our neighbouring stars. The fastest spacecraft humans were able to construct up to this day, Voyager 1, moves away from the Sun at the speed of just 17 kilometres per second. If we wanted to get to the star Proxima Centauri using a similar spacecraft, it would take us nearly 75 thousand years.
If we really want to start contemplating the exploration of at least the closest part of our own galaxy, we need to drastically increase the speed of our cosmic wanderers. For instance, if we were able to create a spaceship traveling at ten percent the speed of light (which is more than 1700 times faster than Voyager 1), we would get to our beloved Proxima in considerably more satisfying 40 years.
But sadly, adventurous journeys to other galaxies may never take place. Even if we miraculously managed to construct a spacecraft moving at nearly the speed of light 7 , a journey towards the Andromeda galaxy would take several million years at least!
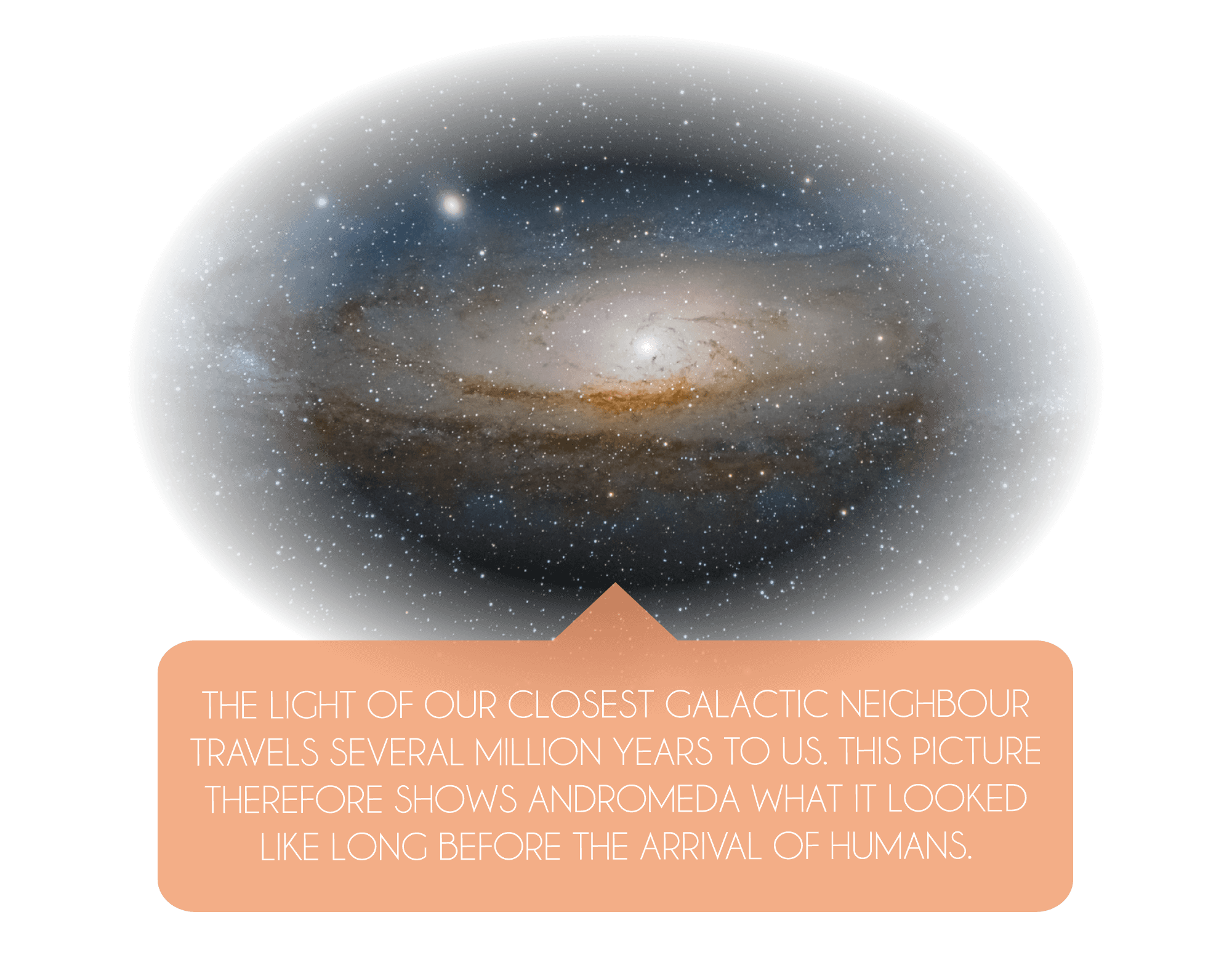
Whether we like it or not, the human race will most likely be trapped inside our minuscule part of the cosmos known as the Milky Way, without the slightest chance of a possibility to explore the wonders of the unbelievably vast cosmos we are all inhabiting.
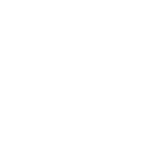
![]() |
![]() |
![]() |
PREVIOUS |
SHARE |
NEXT |
DIVERSE TEMPERATURES OF THE COSMOS
If you have ever been scalded by a hot pot or come into contact with a red-hot stove, then you surely know that it is not a good idea to come into contact with objects of a different temperature than yours. But not everybody knows why.
Temperature is, just like everything else in the universe, just a form of energy. The entire concept of temperature is actually really simple – the greater the temperature of an object, the faster is the motion of the particles from which this object is made. Even atoms and molecules of your body are vibrating furiously and thereby showing their temperature.
And if two objects of different temperatures come into contact, the particles of the object with a higher temperature start bumping into the particles of the second object and therefore giving them their energy until the temperatures of both objects even out. That means that any time you come into contact with a hot object, the temperature of this object starts flowing into your body.
It may not come as a surprise that your body does not appreciate that very much. Its painstakingly made proteins happily use this energy and start altering their structure, which makes them lose their ability to perform the function for which your body has created them in the first place. Basically, you start cooking alive.
But let us go back to the original topic of this chapter – perspective. Imagine that there is an object in front of you and that you start cooling this object gradually. The temperature pilgrimage of the object begins somewhere at room temperature and the velocity of molecules increases gradually. The first milestone it passes is 15 degrees Celsius – the average temperature of our planet. Then it quickly surpasses 0 degrees – the freezing point of water.
The next interesting station is the temperature minus 88 degrees Celsius – the smallest temperature that has ever been measured on Earth. After that, it passes the milestone of 170 degrees below the freezing-point, which is the smallest temperature ever measured on the Moon. Then, the object gets under 220 degrees Celsius – the temperature of the most famous dwarf planet of the solar system, named Pluto.
But before we even know it, our object reaches its final destination. Throughout the whole cooling, the particles of the object have been slowing down constantly – their vibrations have been getting less and less fierce. And eventually, they simply stop. The temperature at which this interesting phenomenon occurs is minus 273.15 degrees Celsius – the smallest possible temperature of the universe, known as the absolute zero. No object can ever cool down under this borderline temperature. 8
The absolute zero is so important, in fact, that a new scale has been created because of it – the Kelvin scale. It is the same as the Celsius scale, only moved by 273.15 degrees. That means that on the Kelvin scale the absolute zero is denoted simply as 0 Kelvin. (And room temperature, for instance, is somewhere around 295 Kelvin).
Let us go back to the very beginning of our objects’ pilgrimage – the room temperature. This time, however, we will go in the opposite direction, on a much interesting journey of the greatest temperatures in the cosmos. If we took our object on a little trip to the middle of the Libyan Desert, with a bit of luck we would be able to heat it up to 58 degrees Celsius – the greatest recorded air temperature here on Earth. However, if you wish to grant your object even higher temperature, and by any chance you have some surplus money (a few billion dollars would do), you may be able to take it to the Moon, whose temperature is sometimes even a hundred degrees Celsius. And once you are on the Moon, why not make a trip to the hottest planet of our solar system – Venus? Its atmosphere it incredibly thick, so it causes a tremendous greenhouse effect. That keeps the temperature of Venus on boiling 400 degrees Celsius.
However, if you do not wish to participate in lengthy space missions, during which your body receives huge doses of cosmic radiation, you can encounter high temperatures even here on Earth – inside the Earth, to be precise. If you miraculously managed to dig a hole several hundred kilometres deep, you would get somewhere near the middle of the Earth’s mantle, whose temperature exceeds an impressive six thousand degrees Celsius.
A similar temperature (about 5500 degrees Celsius) is also present on the surface of our parental star. But that is nothing compared to the gigantic temperatures in the Sun’s heart. If you submerged into the Sun’s plasma and traveled nearly 700 thousand kilometers into the very center, you would encounter temperatures that can reach up to 15 million degrees.
However, if these temperatures still feel unsatisfactory, you can travel many light years to a huge dying star. Once such a star reaches the state of a supernova, it heats to absolutely unprecedented temperatures. The temperature of a supernova explosion often exceeds 50 million degrees. This tremendous temperature causes supernovae to create even very heavy elements, as I have already mentioned in the previous chapter.
But for the highest recorded temperature in the universe, you do not need to go far at all. Just take a small trip to the border between France and Switzerland, where the greatest pride of theoretical physicists is built – the Large Hadron Collider. The task of this ingenious structure is actually very simple – to send accelerated particles against each other through a huge, 27 kilometers long underground tunnel. During these magnificent particle collisions, scientists are trying to simulate the state of the cosmos right after the Big Bang. They are hoping to reveal some of the infinite secrets of the cosmos. In fact, that is exactly what they managed to do back in 2012, when the Higgs boson was discovered with great pomp.
However, we are interested in a different piece of information right now – during collisions in the Large Hadron Collider, particles are heated to monstrous temperatures. The highest temperature reached during one such explosion exceeded staggering 5 trillion degrees. The particles that participated in this explosion can therefore safely claim the prize for by far the greatest temperature we have ever observed in the universe.
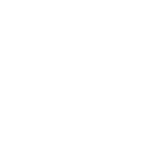
![]() |
![]() |
![]() |
PREVIOUS |
SHARE |
NEXT |